Research Article - The International Tinnitus Journal (2025) Volume 29, Issue 1
The Toll-like-receptors signaling modulated by exosomal miRNA is linked with induction of autophagy for protecting auditory system: Novel therapeutic target for inner ear disease
1Department of Otolaryngology, Head and Neck Surgery, Kawagoe Ear Institute, Keio University School of Medicine, Saitama, Tokyo, Japan
2Department of Otolaryngology-Head and Neck Surgery, Graduate School of Medicine, University of Tokyo, Tokyo, Japan
Send correspondence to: Ken Hayashi Department of Otolaryngology, Head and Neck Surgery, Kawagoe Ear Institute, Keio University School of Medicine, Saitama, Tokyo, Japan, E mail: kenhayashi0811@icloud.com
Paper submitted on Feb 21, 2024; and Accepted on Mar 17, 2024
Citation: Ken Hayashi. The Toll like Receptors Signaling Modulated by Exosomal Mirna is linked with Induction of Autophagy for Protecting Auditory System: Novel Therapeutic Target for Inner Ear Disease. Int Tinnitus J. 2024;29(1): 33-43.
Abstract
Nanoparticle-based drug delivery (30–150 nm) is a promising new strategy for the treatment of inner ear diseases. Exosomes are biological nanoparticles secreted in the extracellular area from all cells, including inner ear sensory cells that mediate intercellular messages to recipient cells by delivering RNAs cargo. There is especially strong evidence that microRNA (miRNA) cargo in extracellular vesicles can alter the function of recipient cells. Therefore, exosomal miRNAs hold great potential for clinical application in the treatment of inner ear diseases. Recently, researchers have identified two fascinating aspects of exosomal miRNAs. First, they are recognized by Toll-like receptors (TLRs), which trigger the signaling cascade of immune responses. Second, they are focused on the autophagic cargo of exosomes and the signaling pathways involved in exosome-induced autophagy in recipient cells. Autophagy is a dynamic lysosomal degradation and recycling process in eukaryotic cells. The induction of autophagy confers protection against inner ear sensory cell death. Thus, exosomal miRNAs that interact with TLRs in sensory hair cells could induce autophagy to protect the auditory system. Extracellular exosomes provide new insights for research with the help of natural nanoparticles, not only as detection indices but also as nanocarriers for the delivery of therapeutics against inner ear diseases. However, this technique has not been established for the auditory system, although it has been well discussed in the field of cancer. In this paper, we review the coordination mechanisms of exosomal miRNAs and autophagy through the TLRs signaling pathway and summarize the function of extracellular exosomes as potential carriers in a novel drug delivery system for inner ear disease.
Introduction
The World Health Organization (WHO) has reported that approximately 466 million people worldwide will experience hearing loss by 2020, and this number will increase to over 34 million people by 2050. The pathogenesis of hearing loss has a wide range, such as genetic, viral infection, autoimmune, microvascular disorder, the use of particular drugs with ototoxicity, exposure to excessive noise and aging. Over 90% of hearing loss is classified as sensorineural, involving loss of inner ear sensory cells or auditory neurons. The WHO warns about the possibility that an increase in hearing loss results in an annual global cost of US$ 750 billion, including nursing care. However, there are currently no effective treatments or preventive methods. The cochlea is surrounded by a bony labyrinth, and the vasculature and fluids of the inner ear are closed by the Blood-Labyrinthine Barrier (BLB). Physical inaccessibility, owing to the structural characteristics of the inner ear, discourages the delivery and effect of drugs to inner ear hair cells. Preserving or treating hearing loss is critical in a super-aging society. Recent studies have shown that extracellular vesicles or exosomes might be effective as new nanocarriers for inner ear disease, as they are secreted from inner ear HCs and incorporated into HCs, crossing the BLB [1-4]. Based on exosome studies in other fields, we believe that exosomes may have pivotal effectiveness as a new treatment for inner ear diseases. In this article, we review the latest research on the importance of exosomes in auditory systems including the cross of the BLB, exosome biogenesis, exosome-activating intracellular signal cascades associated with autophagy and apoptosis regulating cell fate and Toll-Like-Receptors (TLRs), a family of pattern recognition receptors, controlling the innate immunity via miRNAs, and finally shed light to the possibility of miRNA-enriched exosomes as a new treatment for inner ear disease.
The importance of exosomes in hearing system
The auditory system has many signaling pathways, including the Wnt and FGF pathways, which play important roles in the development of the sensory system [5]. Hearing loss mainly causes sensing or loss of auditory hair cells (HCs) and neurons in the inner ear, and is induced by aging, hereditary factors, noise trauma or ototoxic drug [6,7]. Recently, three interesting studies suggested that exosomes exist in the inner ear (tissue and auditory cell lines) and can be released from auditory cells affected by ototoxic drugs [1-4]. Importantly, exosomes can mediate intercellular communication between different cells by delivering exosomal proteins, nucleic acids, and lipids to recipient cells [8]. This suggests that the regulation of exosomes may protect the inner ear from various stresses and prevent the progression of hearing loss. Exosomes can be used as biomarkers of hearing loss or therapeutic targets for sensorineural hearing loss. Another study reported that an exosome (exo)-associated adeno-associated vector (AAV) has been successfully used as a non-toxic and novel gene vector for inner ear HCs (Inner HCs and Outer HCs) in mouse [9]. In this study, approximately 95% of the exo-AAV vector was transduced into both HCs (IHCs and OHCs) in culture medium, and the transduction efficiencies of IHCs and OHCs in vivo were 88 and 25%, respectively, when injected into the mouse cochlea through a round window (RW). Another study indicated that Supporting Cells (SCs) which function as glia-like cells in the inner ear produce HSP70 to protect HCs [10]. The same group recently reported that exosomes derived from SCs were significantly increased by heat shock and could improve the HCs survival rate after exposure to neomycin by interacting with TLR4 receptors on the membrane of HCs [1]. These studies showed that exosomes could be released by various stresses and affect the pathological processes of inner ear disease or sensorineural hearing loss, while transducing as a novel therapeutic tool. We believe that exosomes will make a significant contribution as biomarkers or therapeutic targets for inner ear diseases, including sensorineural hearing loss, in the near future, although there are only a few reports on exosomes in the auditory system. First, we introduce three important studies on exosomes in the inner ear.
Exosomes from inner ears tissues
A study2 by Wong EHC et al reported that extracellular vesicles with the characteristics of exosomes in size range (around 100 nm) and appearance were isolated from primary cultured inner ear cells of rats after treatment with gentamicin and cisplatin, showing different degrees of damage to hair cells (cisplatin: much and gentamicin: little)2. They described that typical exosome markers including HSP 70/71 (Hspa5, Hspa1a, Hspa8) and HSP 90 (Hsp90b1, Hsp90aa1, Hsp90b1, Hsp90ab1) and markers for the organ of Corti and hair cells such as alpha-actinin 1 (Actn 1) and Actn 4, myosin heavy chain (Mh9) and ATP5A1 were detected from exosomes under control and exposure of ototoxic drugs, while the expression of markers associated with SNHL such as myosin heavy chain (Myh 14) and markers associated with congenital SNHL and abnormal ear cartilage like V-type proton ATPase subunit B (V-ATPase B) increased after exposure of ototoxic drugs [11,12]. Interestingly, proteomic analysis of inner ear markers from isolated exosomes detected the following proteins associated with sensorineural hearing loss: transmembrane protein 33 (TMEM33) described in noise-traumatized rat cochleae [13] and phosphoglucomutase 1 (Pgm1) in cisplatin-induced cytotoxicity [14]. Thus, after exposure to cytotoxic drugs, exosomes may reflect cell fate. Tmem33 is usually localized in the endoplasmic reticulum (ER) and nuclear envelope. It is considered that Tmem33 regulate the tubular structure of the ER by interfering with reticulons [15]. These results indicate that exosomes have potential as biomarkers for inner ear diseases. Lipoma HMGIC fusion partner-like 5 (LHFPL5), also known as the tetraspan membrane protein of hair cell stereocilia (TMHS) is an essential component in the mechanical transduction mechanism of OHCs and IHCs. It has been reported that the deletion of LHFPL5 results in hearing loss [16]. Exosomes derived from the inner ear can be detected in different body fluids after exposure to various stress including hypoxia [17], heat shock [1,18], oxidative stress [19] and acidic pH [20]. Interestingly, chaperonin-containing T-complex polypeptide 1 subunit 8 (cct8) was significantly enriched in heat-shocked HCs, but not in heat-shocked SCs using immunohistochemistry or RNA-Seq [21]. Exosomes are basically considered to play important roles in cell-to-cell communication [22] and genetic material exchange [23]. Exosomes derived from the inner ear should have the same function as those derived from other organs. Thus, exosomes can be used as therapeutic targets for inner ear diseases, including SNHL. Supporting this hypothesis, recent studies have described that exosome (exo)-AAV-HA-Lhfpl5 injection through RW could particularly rescue hearing in Lhfpl5-/- mice [9] and exosomes carrying HSP70 derived from heat-shocked utricles improved the survival rate of HCs after exposure to aminoglycoside drugs; on the other hand, the depletion of exosomes from the extracellular environment decreased the effect of heat shock protecting HCs [1].
Exosomes from auditory cell lines under various stress and protein profile
A study [3] by Kalinec GM et al indicated that the original extracellular vesicles (EVs) isolated from auditory cell line, HEI-OC1 cells, were separated by size into two fractions, Small (S)-EVs (diameter ≤150 nm) and Large (L)-EVs (diameter >150 nm). HEI-OC1 was the found to produce more S-EVs than L-EVs, and the protein profile showed that S-EVs fraction was lager and greater in the point of number and diversity [2]. Annexin A1 (ANXA1) and galectins 1 and 3 (Gal-1 and Gal-3) were detected only in the S-EV fraction, whereas cytokines (CSF1, colony-stimulating factor 1) were detected in both S-EVs- and L-EV fractions. The authors suggested that these three molecules (ANXA1, Gal-1, and Gal-3) make S-EVs attractive as potential nanocarriers in auditory cells. In addition, they investigated whether EVs from HEI-OC1 cells can be loaded with pharmacological agents recognized as anti-inflammatory and pro-resolving agents and indicated that lipid metabolites were significantly increased in both of EVs loaded with arachidonic acid (AA), eicosapentaenoic acid (EPA), and docosahexaenoic acid (DHA), which play important roles in cell signaling, including inflammation and immunity, while L-EVs had a more efficient incorporation of asparagin (ASP), 15-HETE to lipoxin A4 (LXA4) and resolvin D1 (RvD1) than S-EVs. This indicates that L-EVs can carry anti-inflammatory and pro-resolving agents more effectively than S-EVs [24].
The crosslink of stress-induced exosomes with autophagy
Autophagy is a lysosomal degradation system for cellular homeostasis that plays a central role in preserving auditory HCs or spiral ganglion neurons (SGNs) from various environmental and cellular stresses [25-27], whereas autophagy impairment results in hearing loss [28-30]. As shown in (Figure 1), 3crosslinking of exosome biogenesis with the autophagy pathway protects cellular homeostasis [31,32]. A previous study reported that autophagy positively regulates exosome secretion through the RhoC/ROCK2 pathway (Rho, Ras homolog gene; ROCK, Rho-associated coiled-coil-forming protein kinase) in rat nucleus pulposus cells [33]. Exosomes contain molecular signals that regulate autophagy, which in turn regulates exosomal activities [34,35]. A recent study suggested that common molecules contribute to the generation of exosomes and autophagic flux [36,37]. Soluble N-ethylmaleimide-sensitive-factor attachment protein receptor (SNARE) facilitates the fusion of Multivesicular Body (MVBs) with the plasma membrane and mediates autophagy-mediated membrane fusion. A previous study showed that membrane fusion is required for the maturation of autophagosomes, depending on the activity of SNARE family proteins such as vesicle-associated membrane protein 7 (VAMP7), syntaxin 7, and 8 [38].
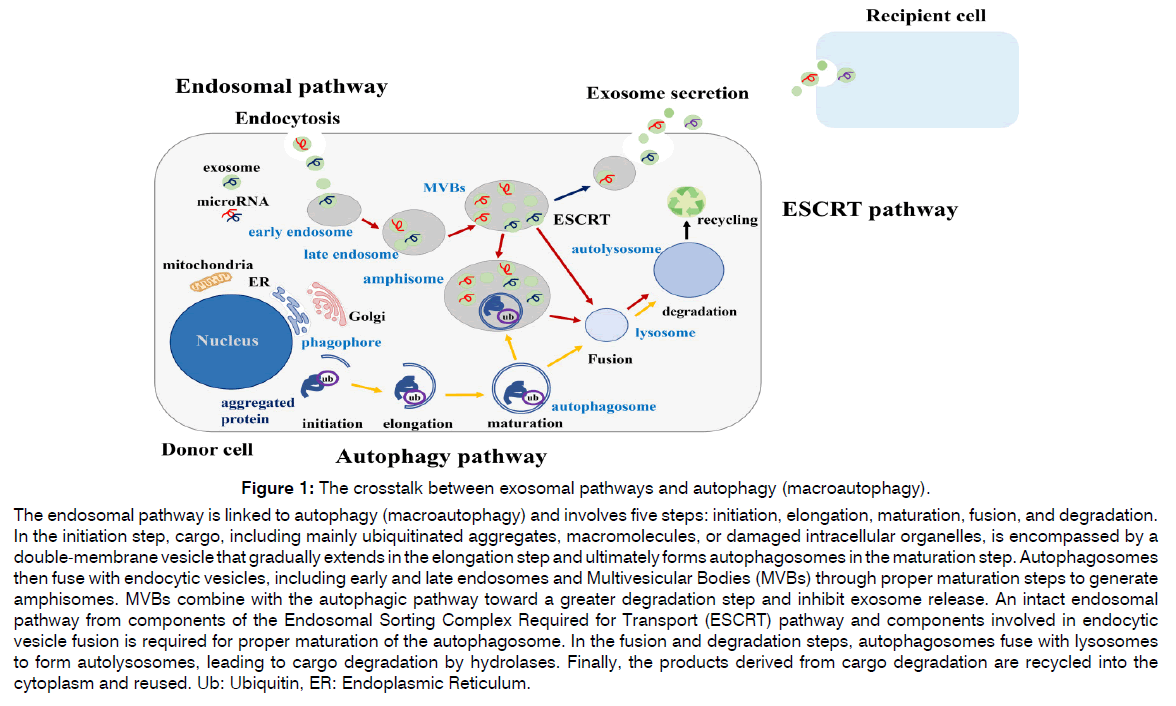
Figure 1: The crosstalk between exosomal pathways and autophagy (macroautophagy).
The endosomal pathway is linked to autophagy (macroautophagy) and involves five steps: initiation, elongation, maturation, fusion, and degradation.
In the initiation step, cargo, including mainly ubiquitinated aggregates, macromolecules, or damaged intracellular organelles, is encompassed by a
double-membrane vesicle that gradually extends in the elongation step and ultimately forms autophagosomes in the maturation step. Autophagosomes
then fuse with endocytic vesicles, including early and late endosomes and Multivesicular Bodies (MVBs) through proper maturation steps to generate
amphisomes. MVBs combine with the autophagic pathway toward a greater degradation step and inhibit exosome release. An intact endosomal
pathway from components of the Endosomal Sorting Complex Required for Transport (ESCRT) pathway and components involved in endocytic
vesicle fusion is required for proper maturation of the autophagosome. In the fusion and degradation steps, autophagosomes fuse with lysosomes
to form autolysosomes, leading to cargo degradation by hydrolases. Finally, the products derived from cargo degradation are recycled into the
cytoplasm and reused. Ub: Ubiquitin, ER: Endoplasmic Reticulum.
SNAREs initiate crosslinking of autophagosome with exosomes [39]. Furthermore, Rab11, an MVB-associated protein, acts as an initiator of the assembly of autophagy-related (ATG) proteins that form autophagosomes [40]. A previous report suggested that ALG-2 interacting protein X (ALIX), an exosome marker, mediates the discrepancy between lysosomal degradation and exosomal secretory pathways as an exosomal cargo [41,42], while the inhibition of ALIX fundamentally decreases the induction of autophagy, modulating the crosslink arrangement between exosome biogenesis and the autophagy pathway [43]. ATG12–ATG3 complex regulates MVB shape, distribution of late endosomes, and eventually exosome biogenesis. Autophagy-related (ATG) proteins have been reported to play pivotal roles in exosome biogenesis under normal and pathological conditions [44]. Researchers presented that ATG5 mediates the detachment of vacuolar proton pumps (v-ATPases) from MVBs, inhibiting the acidic environment of the MVBs lumen and directing MVBs to the PM instead of lysosomes. These results indicate that the MVB lumen pH is a determinant of exosome fate. Another study suggested that ATG16L1 and ATG5 contribute to the secretory pathway, protecting exosomes from the degradation pathway, and that the ATG12–ATG3 complex facilitating LC3 conjugation plays an important role in exosome biogenesis via interaction with ALIX, a protein that interacts with the ESCRT machinery to produce ILVs [43].
Exosomal secretion via autophagy regulators
Exosomes are considered an important tool for the induction of autophagic flux in target cells by transferring autophagic activators or autophagy-related molecules [36]. Autophagy is activated in recipient cells after the internalization of exosomes [45]. A recent report suggested that EVs derived from mesenchymal stem cells (MSCs) contain several mRNAs of autophagy-related proteins, including Beclin-1, LC3, and ATG7, which increase autophagy flux in hematopoietic stem cells [46]. These findings provide novel evidence that exosomes regulate autophagy by transferring autophagic components through autophagic regulators.
Cross-regulation by toll-like receptor-ligand signal pathway and exosomal miRNAs mediating cell-cell communication
MiRNAs are one of the non-coding RNA families binding to the 3’ untranslated regions of their target messenger RNAs (mRNAs) for suppressing translation or degradation of the mRNAs [47,48], and have a pivotal role in many biological processes including autophagy, apoptosis, senescence or inflammation. Therefore, the dysregulation of miRNAs has been reported to be associated with pathogenesis or progression of multiple human diseases including hearing loss [49,50]. Recent evidence has demonstrated that extracellular vesicles, such as exosomes and shed microvesicles isolated from different cell types, may be novel mediators of cell-cell communication, including mRNAs, miRNAs, long non-coding RNAs, lipids, and DNA fragments. These active cargo molecules are packaged and released into exosome-derived cells and taken up by neighboring cells, where they are functionally active [51,52]. miRNAs are ubiquitously expressed in exosomes and are involved in the modulation of the host immune response, expression of some activated molecules, enhanced tumor cell invasion, and mediation of intercellular communication [53].
Interestingly, recent studies have demonstrated that several miRNAs, including miR-21, miR-146, miR-155, and let-7 family, regulate the transcriptional responses of Toll-like Receptors (TLRs) expressed in the inner ear, membrane passing proteins [54,55] or target proteins in TLR signaling pathways involved in the regulation of various processes, such as inflammation, aging, cellular infiltration, and immunity development [56,57]. Some miRNAs function as TLRs ligands, activating TLRs signaling pathways involved in intercellular communication in various microenvironment [58,59].
The relationship between autophagy and TLRs
TLRs also function as autophagy mediators. Previous reports have suggested that TLRs have a regulatory cross-talk with autophagy [60,61], that TLR9 translocates from the ER to lysosomes upon autophagy induction in the fusion step of the autophagy process, and that the TLR9-mediated lysosomal cargo response sustains autophagy flux [62]. Another study indicated that TLR2 induced autophagy in an acute kidney injury model induced by cisplatin and improved global renal tissue parameters [63]. The expression of TLR2 and TLR4 was increased in hippocampal neurons of epileptic mice, while autophagy was inhibited in hippocampal neurons of epilepsy mice over-expressing MiR-421, linking with the TLR/MYD88 signaling pathway [64]. Importantly, exosomes from neutrophils infected with Mycobacterium tuberculosis contain TLR2/6 ligands that induce autophagy flux in macrophages [65]. TLR7 has two binding sites for small ligands and single-stranded RNA (ssRNA), including miRNA, and is activated by guanosine or ssRNA [66](Figure 2). Based on these previous reports, we confirmed that exosomal let-7b increased the expression of LC3-II in auditory cells, inducing the activation of TLR7 (Figure S1). This result indicated that exosomal let-7b mediates the induction of autophagy by TLR7 ligands (Figure 2).
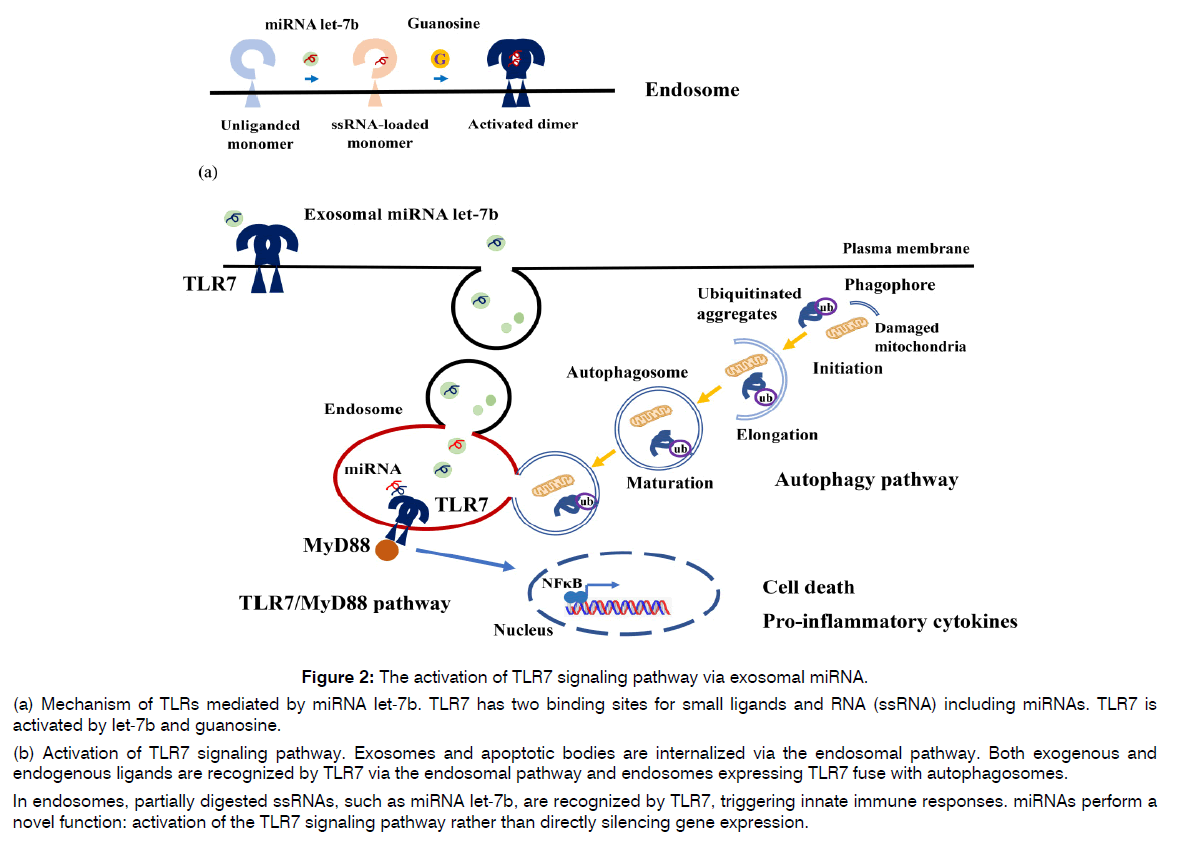
Figure 2: The activation of TLR7 signaling pathway via exosomal miRNA.
(a) Mechanism of TLRs mediated by miRNA let-7b. TLR7 has two binding sites for small ligands and RNA (ssRNA) including miRNAs. TLR7 is activated by let-7b and guanosine.
(b) Activation of TLR7 signaling pathway. Exosomes and apoptotic bodies are internalized via the endosomal pathway. Both exogenous and endogenous ligands are recognized by TLR7 via the endosomal pathway and endosomes expressing TLR7 fuse with autophagosomes.
In endosomes, partially digested ssRNAs, such as miRNA let-7b, are recognized by TLR7, triggering innate immune responses. miRNAs perform a novel function: activation of the TLR7 signaling pathway rather than directly silencing gene expression.
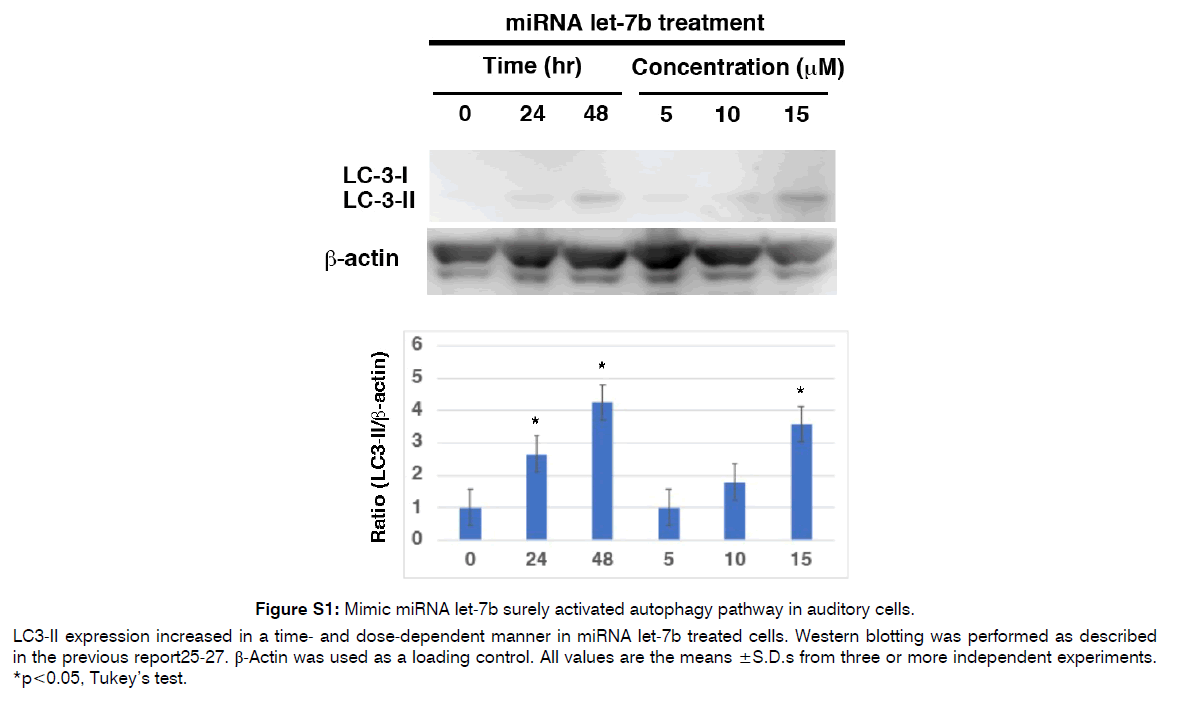
Figure S1: Mimic miRNA let-7b surely activated autophagy pathway in auditory cells.
LC3-II expression increased in a time- and dose-dependent manner in miRNA let-7b treated cells. Western blotting was performed as described in the previous report25-27. β-Actin was used as a loading control. All values are the means ±S.D.s from three or more independent experiments. *p<0.05, Tukey’s test.
Exosomes could cross blood labyrinth barrier and blood brain barrier
One of the biggest problems of drug delivery to the inner ear via the vasculature for inner ear disease is that BLB restricts effective delivery as a barrier between the vasculature and fluids of the inner ear, as it is separated from the systemic circulation by BLB [67,68]. We review the structure of the BLB and BBB and introduce how to cross the BLB using exosomes for the treatment of inner ear diseases based on a previous report [4]. The capillaries and cell types that form the BLB and BBB have many common structural features. The endothelium of the BLB is surrounded by a basement membrane in pericytes but does not have an outer basement membrane, similar to the BBB. Tight junctions between endothelial cells in the BBB and BLB are composed of similar proteins. Perivascular resident macrophages (PVM/Ms), a hybrid cell type with the characteristics of both macrophages and melanocytes, are wrapped around the basement membrane surrounding the BLB endothelium. In contrast, the endothelium of the BBB is surrounded by a basement membrane that is split to wrap pericytes, sharing the Basement Membrane (BM1) with pericytes. The endfeet of astrocytes form a second Basement Membrane (BM2), creating a perivascular space between the two basement membranes, covering most of the outer basement membrane, and encapsulating the BBB capillaries within the CNS.
How does EVs cross the blood labyrinth barrier and blood brain barrier?
A previous report showed that there are five routes for exosomes interacting with a receiving cell4: (1) association with a protein G-coupled receptor on the cell surface stimulating a signaling cascade, (2) fusion to the cell surface and capture of the exosome content in the cytoplasm, leading to the activation of cell signaling cascades, (3) micropinocytosis, (4) nonspecific or lipid rafts, and (5) receptor-mediated transcytosis, leading to the activation of the endocytosis pathway and storage in the MVB. Then, three intracellular events for exosomes occur in the cytoplasm: (1) degradation by lysosomes, (2) signal induction through back fusion in the MVB, releasing its contents in the cytoplasm, or (3) trafficking from the MVB to the plasma membrane in the receiving cell. Exosomes can cross the BLB or BBB because there are melting pots for exosomes as described here [69].
Circulation EVs can cross the blood labyrinth barrier and blood brain barrier
We believe that exosomes may be highly useful nanocarriers for delivering therapeutics to protect the inner ear from various stresses, as described in this review. Exosomes have many advantages as nanocarriers in terms of packaging capacity, biological compatibility, and the ability to penetrate the blood-labyrinth barrier or blood-brain barrier compared to other delivery systems, including gene therapy by virus or non-virus [70]. Exosomes are used as a therapeutic delivery tool for proteins, nucleic acids, and small molecules in the various area [71]. Interestingly, the designed cargo can also be delivered to the brain following the systemic administration of targeted exosomes [72]. This result suggests that almost the same methods could be useful for the treatment of inner ear diseases because the BLB has many similarities with the BBB. It has been reported that the complement of integrins on exosomes determines the tissue affinity [73]. An inner ear researcher indicated that the heterodimers between integrin subunits (α-3, α-7, α-V, and β-1) might point exosomes on specific inner ear cell types [1]. A previous report demonstrated that conventional AAV1-GFP transduced some IHCs and a few OHCs; the transduction rate using conventional AAV1 was as follows: cochleostomy delivery, 36% GFP-positive IHCs and 17% GFP-positive OHCs; round window membrane (RWM) injection, 65% GFP-positive IHCs and 14% OHCs. On the other hand, the transduction rate using exo-AAV1-GFP vectors was as follows: cochleostomy, 63% of IHCs and 28% of OHCs; RWM injection, 88% of IHCs and 25% of OHCs. This result suggests that exosome-associated adeno-associated virus (AAV) is a more effective nanocarrier for IHCs and OHCs than conventional AAV9. Another study reported that HSP70, a critical component of the protein cargo of utricle-derived exosomes, may act as a hair cell-protective exosome in vitro1. Taken together, exosomes can protect sensory HCs from various stresses by delivering therapeutic molecules, thereby reversing hearing loss.
Delivery techniques miRNA-enriched extracellular vesicles
As described in (Figure 3), the delivery of EVs into IHCs and OHCs could become a pivotal treatment for inner ear diseases because EVs can cross the BLB and BBB and encapsulate miRNAs. Indeed, miRNAs (or siRNAs) have been used as therapeutic molecules in EVs to treat neurodegenerative diseases and cancer [74]. This is because miRNAs can travel long distances in body fluids or blood and function normally in recipient cells, as they can maintain high stability when encapsulated in EVs. Enriched miRNAs in EVs may be good initiators for EV-based therapies for the inner ear. Finally, we described the benefits and techniques of EV-miRNA therapies as key challenges for the treatment of inner ear diseases in the near future, although their use in actual clinical settings remains difficult. Two approaches have been introduced for synthesizing miRNA-enriched EVs now [75]. The first involves producing a cell line that exhibits a high concentration of targeted miRNAs in the cytosol. Second one is to isolate EVs from the culture medium (cell lines or tissues) or body fluids and enclose them with the targeting miRNA using established methods [76]. Based on this principle, we believe that the same techniques for miRNA-enriched EV therapies should be applied to protect inner ear HCs from various stresses, and that the immortalized auditory cell line HEI-OC1 would be useful for this therapy [2]. In addition, targeted miRNA inhibitors or miRNA mimics can be loaded into EVs using established methods. We confirmed that exposure to mimic miRNA let-7b or TLR7 agonist (loxoribine) decreased cell viability in HEI-OC1 cells, while cell viability remained unchanged when the cells were simultaneously exposed to the mimic RNA let-7b inhibitor and loxoribine (Figure S2). This result suggests that a mimic miRNA let-7b inhibitor suppress TLR 7-mediated auditory cell death and has an auditory cytoprotective effect. We consider that EV-miRNA or miRNA inhibitors with GUGU-rich motifs, such as let-7b, can effectively be enclosed in inner ear HCs through TLRs, and that EV-miRNA derived from autophagy induced by various stresses might have protective effects on the inner ear from our unpublished data. Another benefit of miRNA-enriched EVs is their stability under normal physiological conditions. Another study reported that EV-miRNAs can remain stable in a physiological environment for 1.5–13 h based on the results from different miRNAs (let-7, miR-1, miR-206, miR-233, and miR-133a) with different GC contents [77]. We found that TLR7 expression was time-dependently induced 48 h after exposure to the mimic miRNA let-7b in HEI-OC1 cells (Figure S3). This indicated the stability of the mimic miRNA let-7b.
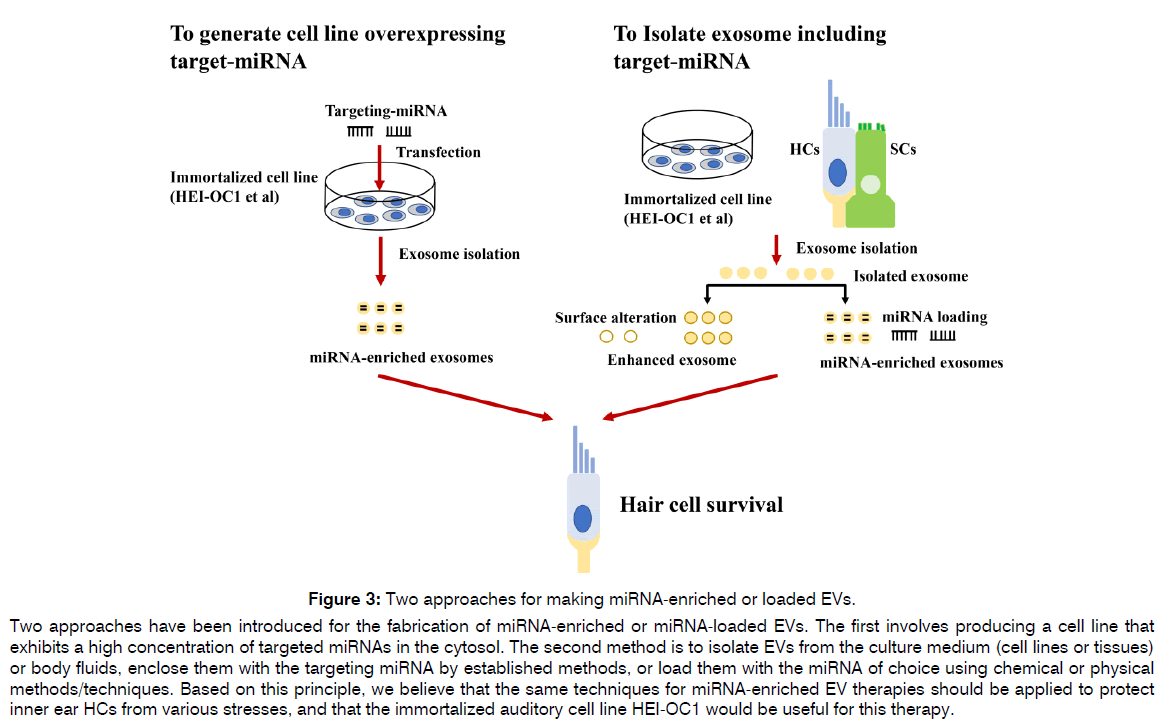
Figure 3: Two approaches for making miRNA-enriched or loaded EVs.
Two approaches have been introduced for the fabrication of miRNA-enriched or miRNA-loaded EVs. The first involves producing a cell line that exhibits a high concentration of targeted miRNAs in the cytosol. The second method is to isolate EVs from the culture medium (cell lines or tissues) or body fluids, enclose them with the targeting miRNA by established methods, or load them with the miRNA of choice using chemical or physical methods/techniques. Based on this principle, we believe that the same techniques for miRNA-enriched EV therapies should be applied to protect inner ear HCs from various stresses, and that the immortalized auditory cell line HEI-OC1 would be useful for this therapy.
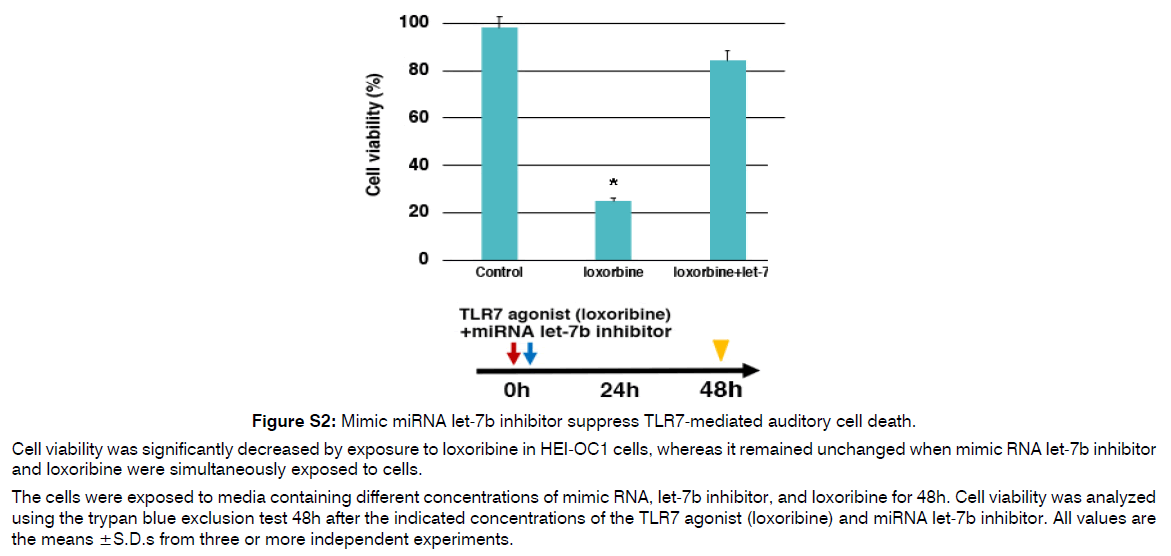
Figure S2: Mimic miRNA let-7b inhibitor suppress TLR7-mediated auditory cell death.
Cell viability was significantly decreased by exposure to loxoribine in HEI-OC1 cells, whereas it remained unchanged when mimic RNA let-7b inhibitor and loxoribine were simultaneously exposed to cells.
The cells were exposed to media containing different concentrations of mimic RNA, let-7b inhibitor, and loxoribine for 48h. Cell viability was analyzed using the trypan blue exclusion test 48h after the indicated concentrations of the TLR7 agonist (loxoribine) and miRNA let-7b inhibitor. All values are the means ±S.D.s from three or more independent experiments.
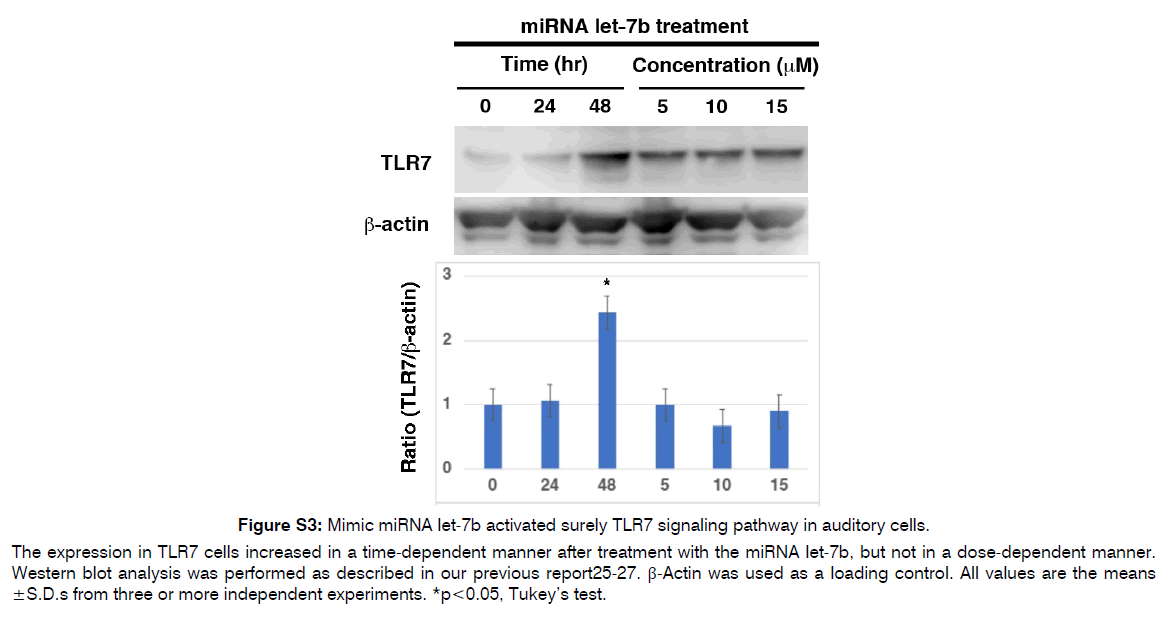
Figure S3: Mimic miRNA let-7b activated surely TLR7 signaling pathway in auditory cells.
The expression in TLR7 cells increased in a time-dependent manner after treatment with the miRNA let-7b, but not in a dose-dependent manner. Western blot analysis was performed as described in our previous report25-27. β-Actin was used as a loading control. All values are the means ± S.D.s from three or more independent experiments. *p<0.05, Tukey’s test.
Conclusion
In this article, we reviewed the coordinate mechanisms for exosomal miRNA and autophagy through the TLRs signaling pathway and the roles of apoptotic vesicles derived from dead cells as the main regulators of the corresponding immune regulation, and summarized the possibility of miRNA-enriched exosomes with the ability to cross the BLB as potential nanocarriers for inner ear disease in the near future. The field of miRNA-enriched EV therapies may expand with the use of immortalized cells, such as auditory cells, as a cell source for capturing EVs in animal studies and even clinical trials. Of course, we know that there is a significant hurdle to overcome for treating inner ear disease with EVs enclosing miRNAs in the clinic. However, we believe that miRNA-enriched EVs therapies have high potential as new treatments for inner ear diseases in terms of effectiveness, safety, and certainty. Further developments in this field are expected.
Conflicts of Interest and Source of Funding
The authors declare no conflict of interest.
References
- Breglio AM, May LA, Barzik M, Welsh NC, Francis SP, Costain TQ, et al. Exosomes mediate sensory hair cell protection in the inner ear. J Clin Invest. 2020;130(5):2657-72.
- Wong EH, Dong YY, Coray M, Cortada M, Levano S, Schmidt A, et al. Inner ear exosomes and their potential use as biomarkers. Plos One. 2018;13(6):e0198029.
- Kalinec GM, Gao L, Cohn W, Whitelegge JP, Faull KF, Kalinec F. Extracellular vesicles from auditory cells as nanocarriers for anti-inflammatory drugs and pro-resolving mediators. Front Cell Neurosci. 2019;13:530.
- Nyberg S, Abbott NJ, Shi X, Steyger PS, Dabdoub A. Delivery of therapeutics to the inner ear: The challenge of the blood-labyrinth barrier. Sci Transl Med. 2019;11(482):eaao0935.
- Żak M, Klis SF, Grolman W. The Wnt and Notch signalling pathways in the developing cochlea: Formation of hair cells and induction of regenerative potential. Int J Dev Neurosci. 2015;47:247-58.
- Brigande JV, Heller S. Quo vadis, hair cell regeneration?. Nat Neurosci. 2009;12(6):679-85.
- Furness DN. Molecular basis of hair cell loss. Cell Tissue Res. 2015;361(1):387-99.
- Colombo M, Raposo G, Théry C. Biogenesis, secretion, and intercellular interactions of exosomes and other extracellular vesicles. Annu Rev Cell Dev Biol. 2014;30(1):255-89.
- György B, Sage C, Indzhykulian AA, Scheffer DI, Brisson AR, Tan S, et al. Rescue of hearing by gene delivery to inner-ear hair cells using exosome-associated AAV. Mol Ther. 2017;25(2):379-91.
- May LA, Kramarenko II, Brandon CS, Voelkel-Johnson C, Roy S, Truong K, et al. Inner ear supporting cells protect hair cells by secreting HSP70. J Clin Invest. 2013;123(8):3577-87.
- Hong CS, Funk S, Muller L, Boyiadzis M, Whiteside TL. Isolation of biologically active and morphologically intact exosomes from plasma of patients with cancer. J Extracell Vesicles. 2016;5(1):29289.
- Muller L, Mitsuhashi M, Simms P, Gooding WE, Whiteside TL. Tumor-derived exosomes regulate expression of immune function-related genes in human T cell subsets. Sci Rep. 2016;6(1):20254.
- Patel M, Hu Z, Bard J, Jamison J, Cai Q, Hu BH. Transcriptome characterization by RNA-Seq reveals the involvement of the complement components in noise-traumatized rat cochleae. J Neurosci. 2013;248:1-6.
- Huang RS, Duan S, Shukla SJ, Kistner EO, Clark TA, Chen TX, et al. Identification of genetic variants contributing to cisplatin-induced cytotoxicity by use of a genomewide approach. Am J Hum Genet. 2007;81(3):427-37.
- Arhatte M, Gunaratne GS, El Boustany C, Kuo IY, Moro C, Duprat F, et al. TMEM33 regulates intracellular calcium homeostasis in renal tubular epithelial cells. Nat Commun. 2019;10(1):2024.
- Xiong W, Grillet N, Elledge HM, Wagner TF, Zhao B, Johnson KR, et al. TMHS is an integral component of the mechanotransduction machinery of cochlear hair cells. J Cell. 2012;151(6):1283-95.
- King HW, Michael MZ, Gleadle JM. Hypoxic enhancement of exosome release by breast cancer cells. BMC Cancer. 2012;12:1-0.
- Clayton A, Turkes A, Navabi H, Mason MD, Tabi Z. Induction of heat shock proteins in B-cell exosomes. J Cell Sci. 2005;118(16):3631-8.
- Hedlund M, Nagaeva O, Kargl D, Baranov V, Mincheva-Nilsson L. Thermal-and oxidative stress causes enhanced release of NKG2D ligand-bearing immunosuppressive exosomes in leukemia/lymphoma T and B cells. Plos One. 2011;6(2):e16899.
- Parolini I, Federici C, Raggi C, Lugini L, Palleschi S, De Milito A, et al. Microenvironmental pH is a key factor for exosome traffic in tumor cells. J Biol Chem. 2009;284(49):34211-22.
- Sadler E, Ryals MM, May LA, Martin D, Welsh N, Boger ET, et al. Cell-Specific transcriptional responses to heat shock in the mouse utricle epithelium. Front Cell Neurosci. 2020;14:123.
- Denzer K, Kleijmeer MJ, Heijnen HF, Stoorvogel W, Geuze HJ. Exosome: from internal vesicle of the multivesicular body to intercellular signaling device. J Cell Sci. 2000;113(19):3365-74.
- Zöller M. Tetraspanins: push and pull in suppressing and promoting metastasis. Nat Rev Cancer. 2009;9(1):40-55.
- Poorani R, Bhatt AN, Dwarakanath BS, Das UN. COX-2, aspirin and metabolism of arachidonic, eicosapentaenoic and docosahexaenoic acids and their physiological and clinical significance. Eur J Pharmacol. 2016;785:116-32.
- Hayashi K, Dan K, Goto F, Tshuchihashi N, Nomura Y, Fujioka M, et al. The autophagy pathway maintained signaling crosstalk with the Keap1–Nrf2 system through p62 in auditory cells under oxidative stress. Cell Signal. 2015;27(2):382-93.
- Tsuchihashi NA, Hayashi K, Dan K, Goto F, Nomura Y, Fujioka M, et al. Autophagy through 4EBP1 and AMPK regulates oxidative stress-induced premature senescence in auditory cells. Oncotarget. 2014;6(6):3644.
- Kishino A, Hayashi K, Hidai C, Masuda T, Nomura Y, Oshima T. XBP1-FoxO1 interaction regulates ER stress-induced autophagy in auditory cells. Sci Rep. 2017;7(1):4442.
- Hayashi K, Suzuki Y, Fujimoto C, Kanzaki S. Molecular mechanisms and biological functions of autophagy for genetics of hearing impairment. Gene. 2020;11(11):1331.
- Fujimoto C, Iwasaki S, Urata S, Morishita H, Sakamaki Y, Fujioka M, et al. Autophagy is essential for hearing in mice. Cell Death Dis. 2017;8(5):e2780.
- de Iriarte Rodríguez R, Pulido S, Rodríguez-de la Rosa L, Magarinos M, Varela-Nieto I. Age-regulated function of autophagy in the mouse inner ear. Hear Res. 2015;330:39-50.
- Zheng Y, Hasan A, Babadaei MM, Behzadi E, Nouri M, Sharifi M, et al. Exosomes: Multiple-targeted multifunctional biological nanoparticles in the diagnosis, drug delivery, and imaging of cancer cells. Biomed Pharmacother. 2020;129:110442.
- Gozuacik D, Akkoc Y, Ozturk DG, Kocak M. Autophagy-regulating microRNAs and cancer. Front Oncol. 2017;7:65.
- Hu SQ, Zhang QC, Meng QB, Hu AN, Zou JP, Li XL. Autophagy regulates exosome secretion in rat nucleus pulposus cells via the RhoC/ROCK2 pathway. Exp Cell Res. 2020;395(2):112239.
- Abdulrahman BA, Abdelaziz DH, Schatzl HM. Autophagy regulates exosomal release of prions in neuronal cells. J Biol Chem. 2018;293(23):8956-68.
- Ojha CR, Lapierre J, Rodriguez M, Dever SM, Zadeh MA, DeMarino C, et al. Interplay between autophagy, exosomes and HIV-1 associated neurological disorders: new insights for diagnosis and therapeutic applications. J Viruses. 2017;9(7):176.
- Hassanpour M, Rezabakhsh A, Rezaie J, Nouri M, Rahbarghazi R. Exosomal cargos modulate autophagy in recipient cells via different signaling pathways. Cell Biosci. 2020;10:1-6.
- Desdín-Micó G, Mittelbrunn M. Role of exosomes in the protection of cellular homeostasis. Cell Adhes Migr. 2017;11(2):127-34.
- Nair U, Jotwani A, Geng J, Gammoh N, Richerson D, Yen WL, et al. SNARE proteins are required for macroautophagy. J Cell. 2011;146(2):290-302.
- Fader CM, Sánchez DG, Mestre MB, Colombo MI. TI-VAMP/VAMP7 and VAMP3/cellubrevin: two v-SNARE proteins involved in specific steps of the autophagy/multivesicular body pathways. Biochim Biophys Acta Mol Cell Res. 2009;1793(12):1901-16.
- Puri C, Vicinanza M, Ashkenazi A, Gratian MJ, Zhang Q, Bento CF, et al. The RAB11A-positive compartment is a primary platform for autophagosome assembly mediated by WIPI2 recognition of PI3P-RAB11A. Dev Cell. 2018;45(1):114-31.
- Baietti MF, Zhang Z, Mortier E, Melchior A, Degeest G, Geeraerts A, et al. Syndecan–syntenin–ALIX regulates the biogenesis of exosomes. Nat Cell Biol. 2012;14(7):677-85.
- Hurley JH, Odorizzi G. Get on the exosome bus with ALIX. Nat Cell Biol. 2012;14(7):654-5.
- Murrow L, Malhotra R, Debnath J. ATG12–ATG3 interacts with Alix to promote basal autophagic flux and late endosome function. Nat Cell Biol. 2015;17(3):300-10.
- Guo H, Chitiprolu M, Roncevic L, Javalet C, Hemming FJ, Trung MT, et al. Atg5 disassociates the V1V0-ATPase to promote exosome production and tumor metastasis independent of canonical macroautophagy. Dev Cell. 2017;43(6):716-30.
- Dutta S, Warshall C, Bandyopadhyay C, Dutta D, Chandran B. Interactions between exosomes from breast cancer cells and primary mammary epithelial cells leads to generation of reactive oxygen species which induce DNA damage response, stabilization of p53 and autophagy in epithelial cells. Plos One. 2014;9(5):e97580.
- Kulkarni R, Bajaj M, Ghode S, Jalnapurkar S, Limaye L, Kale VP. Intercellular transfer of microvesicles from young mesenchymal stromal cells rejuvenates aged murine hematopoietic stem cells. Stem Cell Res J. 2018;36(3):420-33.
- Bayraktar R, Van Roosbroeck K, Calin GA. Cell‐to‐cell communication: microRNAs as hormones. Mol Oncol. 2017;11(12):1673-86.
- Calin GA, Sevignani C, Dumitru CD, Hyslop T, Noch E, Yendamuri S, et al. Human microRNA genes are frequently located at fragile sites and genomic regions involved in cancers. Proc Natl Acad Sci. 2004;101(9):2999-3004.
- Mittal R, Liu G, Polineni SP, Bencie N, Yan D, Liu XZ. Role of microRNAs in inner ear development and hearing loss. Gene. 2019;686:49-55.
- Bayraktar R, Pichler M, Kanlikilicer P, Ivan C, Bayraktar E, Kahraman N, et al. MicroRNA 603 acts as a tumor suppressor and inhibits triple-negative breast cancer tumorigenesis by targeting elongation factor 2 kinase. Oncotarget. 2016;8(7):11641.
- Alvarez-Garcia I, Miska EA. MicroRNA functions in animal development and human disease. Development. 2005;132(21):4653–62.
- Valadi H, Ekström K, Bossios A, Sjöstrand M, Lee JJ, Lötvall JO. Exosome-mediated transfer of mRNAs and microRNAs is a novel mechanism of genetic exchange between cells. Nat Cell Biol. 2007;9(6):654-9.
- H. Rashed M, Bayraktar E, K. Helal G, Abd-Ellah MF, Amero P, Chavez-Reyes A, et al. Exosomes: from garbage bins to promising therapeutic targets. Int J Mol Sci. 2017;18(3):538.
- Liew FY, Xu D, Brint EK, O'Neill LA. Negative regulation of toll-like receptor-mediated immune responses. Nat Rev Immunol. 2005;5(6):446-58.
- Liu Y, Yin H, Zhao M, Lu Q. TLR2 and TLR4 in autoimmune diseases: a comprehensive review. Clin Rev Allergy Immunol. 2014;47:136-47.
- O'connell RM, Rao DS, Chaudhuri AA, Baltimore D. Physiological and pathological roles for microRNAs in the immune system. Nat Rev Immunol. 2010;10(2):111-22.
- O'neill LA, Sheedy FJ, McCoy CE. MicroRNAs: the fine-tuners of Toll-like receptor signalling. Nat Rev Immunol. 2011;11(3):163-75.
- Bayraktar R, Bertilaccio MT, Calin GA. The interaction between two worlds: microRNAs and toll-like receptors. Front Immunol. 2019;10:1053.
- Bosisio D, Gianello V, Salvi V, Sozzani S. Extracellular miRNAs as activators of innate immune receptors. Cancer Letters. 2019;452:59-65.
- Delgado MA, Elmaoued RA, Davis AS, Kyei G, Deretic V. Toll‐like receptors control autophagy. EMBO J. 2008;27(7):1110-21.
- Muzes G, Constantinovits M, Furi I, Tulassay Z, Sipos F. Interaction of Autophagy and Toll-Like Receptors: A Regulatory Cross-Talk-Even in Cancer Cells?. Curr Drug Targets. 2014;15(8):743-52.
- De Leo MG, Staiano L, Vicinanza M, Luciani A, Carissimo A, Mutarelli M, et al. Autophagosome–lysosome fusion triggers a lysosomal response mediated by TLR9 and controlled by OCRL. Nat Cell Biol. 2016;18(8):839-50.
- Andrade-Silva M, Cenedeze MA, Perandini LA, Felizardo RJ, Watanabe IK, Agudelo JS, et al. TLR2 and TLR4 play opposite role in autophagy associated with cisplatin-induced acute kidney injury. Clin Sci. 2018;132(16):1725-39.
- Wen X, Han XR, Wang YJ, Wang S, Shen M, Zhang ZF, et al. Retracted: MicroRNA‐421 suppresses the apoptosis and autophagy of hippocampal neurons in epilepsy mice model by inhibition of the TLR/MYD88 pathway. J Cell Physiol. 2018;233(9):7022-7034.
- Alvarez-Jiménez VD, Leyva-Paredes K, García-Martínez M, Vázquez-Flores L, García-Paredes VG, Campillo-Navarro M, et al. Extracellular vesicles released from Mycobacterium tuberculosis-infected neutrophils promote macrophage autophagy and decrease intracellular mycobacterial survival. Front Immunol. 2018 Feb 19;9:272.
- Zhang Z, Ohto U, Shibata T, Krayukhina E, Taoka M, Yamauchi Y, et al. Structural analysis reveals that toll-like receptor 7 is a dual receptor for guanosine and single-stranded RNA. Immunity. 2016;45(4):737-48.
- Neng L, Zhang F, Kachelmeier A, Shi X. Endothelial cell, pericyte, and perivascular resident macrophage-type melanocyte interactions regulate cochlear intrastrial fluid-blood barrier permeability. J Assoc Res Otolaryngol. 2013;14:175-85.
- Zhang W, Dai M, Fridberger A, Hassan A, DeGagne J, Neng L, et al. Perivascular-resident macrophage-like melanocytes in the inner ear are essential for the integrity of the intrastrial fluid–blood barrier. Proc Natl Acad Sci. 2012;109(26):10388-93.
- Chen CC, Liu L, Ma F, Wong CW, Guo XE, Chacko JV, et al. Elucidation of exosome migration across the blood–brain barrier model in vitro. Cell Mol Bioeng. 2016;9:509-29.
- El Andaloussi S, Mäger I, Breakefield XO, Wood MJ. Extracellular vesicles: biology and emerging therapeutic opportunities. Nat Rev Drug Discov. 2013;12(5):347-57.
- Batrakova EV, Kim MS. Using exosomes, naturally-equipped nanocarriers, for drug delivery. J Control Release. 2015;219:396-405.
- Alvarez-Erviti L, Seow Y, Yin H, Betts C, Lakhal S, Wood MJ. Delivery of siRNA to the mouse brain by systemic injection of targeted exosomes. Nat Biotechnol. 2011;29(4):341-5.
- Hoshino A, Costa-Silva B, Shen TL, Rodrigues G, Hashimoto A, Tesic Mark M, et al. Tumour exosome integrins determine organotropic metastasis. Nat. 2015;527(7578):329-35.
- Wiklander OP, Brennan MÁ, Lötvall J, Breakefield XO, El Andaloussi S. Advances in therapeutic applications of extracellular vesicles. Sci Transl Med. 2019;11(492):eaav8521.
- Munir J, Yoon JK, Ryu S. Therapeutic miRNA-enriched extracellular vesicles: current approaches and future prospects. Cell. 2020;9(10):2271.
- Griswold MD, Oatley JM. Concise review: defining characteristics of mammalian spermatogenic stem cells. Stem Cells Int. 2013;31(1):8-11.
- Coenen-Stass AM, Pauwels MJ, Hanson B, Martin Perez C, Conceição M, Wood MJ, et al. Extracellular microRNAs exhibit sequence-dependent stability and cellular release kinetics. RNA Biol. 2019;16(5):696-706.