Malaria, initiated inevitably by examining those apicomplexan parasites Plasmodium, is a huge purpose behind horribleness likewise mortal adversary all around the world particularly in developing countries. The current study aimed to investigate the mice intestinal oxidative damage and mucin regulated gene response to Plasmodium chabaudi infection. The infection of female C57BL/6 mice with 106 P. chabaudi parasitized erythrocytes induced a maximum parasitemia (about 47 %) on day 8 p.i.. Histological examination of stained sections showed some defects in jejunal tissue of mice infected with P. chabaudi were injured and contained marked inflammatory cells. In addition, examination of Alcian blue stained sections demonstrated a significant increase in the number of goblet cells of the jejunal villi. Moreover, the infection induced a significant increase in the level of NO and MDA while the level of GSH was significantly decreased by the infection. Upon infection with P. chabaudi, there was a significant increase in the mRNA expression of MUC2 and MUC4. Based on our results, we can conclude that, the intestinal response to P. chabaudi infection could help in understanding the process of intestinal oxidative damage as well as the role of mucin related genes during infection. Further studies are required to know the mechanism and the pathway by which the parasite induce these intestinal alteration
Keywords |
Intestine, Mice, Plasmodium chabaudi, Oxidative stress, Goblet cells |
Introduction |
Malaria is a dangerous disease that is transmitted to
people through the insect bite of infected mosquitoes with
plasmodium [1]. World health organization reported that,
about 3.4 billion people live in areas at risk of malaria
transmission in 106 countries and territories. In 2013, it
was reported that malaria caused 198 million clinical
episodes, and 500,000 deaths [2]. |
The intestine is not directly affected by malaria, although
abdominal pains and nausea are symptoms of malaria.
This may be due to the infection induced fever. During
the infection of human with Plasmodium, the capillaries
of the intestinal villi could be blocked with parasitized
erythrocytes [3] and are associated with gastrointestinal
injury with defects in metabolism [4,5]. |
The cellular constituents of the innate defense system in
the intestine include epithelial cells, goblet cells, dendritic
cells and macrophages. The front line of this system is the
mucous layer containing goblet cells which secrete mucin
[6,7]. The response of goblet cells was observed in several
intestinal infections due to bacteria, viruses and parasites. Decreases number of goblets cells (hyperplasia) was
observed in some parasitic infections like helminthes [8,9]
but hypoplasia of these cells were observed in coccidial
infection with Eimeria [10,11]. |
Due to infection with Plasmodium parasites, the host
natural immune response is activated and generates large
amounts of reactive oxygen species causing disorganization
between oxidizing species and antioxidants [12]. This
disorganization could lead to a status of oxidative stress.
This induced oxidative stress is considered to be an
important mechanism as a host response to infection that
induces parasite death. Our study aimed to investigate the
intestinal oxidative damage and mucin regulated gene
response to Plasmodium chabaudi infection. |
Materials and Methods |
Animals |
Twenty adult female C57Bl/6 mice weighed 23-27 g and
aged 9-12 weeks were obtained from the animal facilities
of King Saud University, Riyadh, Saudi Arabia. The mice
were bred under specified pathogen-free conditions and fed a standard diet and water ad libitum. The experiments
were approved by state authorities and followed Saudi
Arabian rules for animal protection. |
Infection of Mice |
Blood stages of P. chabaudi were weekly passaged in
Swiss albino mice. Experimental animals were challenged
with 106 P. chabaudi-parasitized erythrocytes. Parasitemia
was evaluated in Giemsa stained blood smears, and total
erythrocytes were counted in a Neubauer chamber. |
Animals were divided into two groups. The first group
served as a vehicle control. The second group was infected
with 106 P. chabaudi-parasitized erythrocytes. All mice
were sacrificed on day 8 post-infection. |
Histological Analysis |
Pieces of jejunum were freshly prepared from mice
on day 8 postinfection with P. chabaudi, fixed in 10%
neutral buffered formalin, and then embedded in paraffin.
Sections were cut and then stained with hematoxylin and
eosin. According to Dommels et al. [13], tissue sections
were scored for inflammatory lesions (infiltrations
by mononuclear cells, neutrophils, eosinophils, and
plasmacytes, for fibrin exudation and lymphangiectasis,
for tissue destruction (enterocyte loss, ballooning
degeneration, edema, and mucosal atrophy), and for
tissue repair (hyperplasia, angiogenesis, granulomas,
and fibrosis). A rating score between 0 (no change from
normal tissue) and 3 (lesions involved most areas and
all the layers of the intestinal section including mucosa,
muscle, and omental fat) was given for each aspect of
inflammatory lesion, tissue destruction, and tissue repair.
The sum of inflammatory lesions, tissue destruction,
and tissue repair scores was used to represent the total
histological injury score (HIS) for each intestinal section.
The sum of the inflammatory lesions was multiplied by 2
to give more weight to this value since the tissue changes
were mainly characterized by inflammatory lesions
[13]. Stained tissue sections were imaged using light
microscope (Olympus, Japan) provided with digital high
resolution camera. |
The number of Goblet Cells |
Sections were stained with Alcian blue for determination of
the goblet cells. For each animal, the number of goblet cells
in the jejunum was counted on at least ten well-orientated
villous-crypt units (VCU). Results were expressed as the
mean number of goblet cells per ten VCU [14]. |
Oxidative Stress |
Part of the jejunum was weighed and homogenized
immediately in order to prepare a 50% (w/v) homogenate
in an ice cold medium containing 50 mM Tris–HCl and
300 mM sucrose. The initial homogenate was centrifuged
at 500 × g for 10 min at 4°C. The supernatant was diluted
with the Tris sucrose buffer to give 10% and was then used
for the various biochemical determinations. |
Glutathione |
Glutathione (GSH) was determined chemically in
jejunal homogenate using Ellman’s reagent [15]. The
method is based on the reduction of Ellman’s reagent
(5,5dithiobis(2-nitrobenzoic acid) with GSH to produce a
yellow compound. The chromogen is directly proportional
to GSH concentration, and its absorbance was measured
at 405 nm. |
Lipid Peroxidation |
Lipid peroxidation in jejunal homogenate were determined
according to the method of Ohkawa et al. [16] by using 1 ml
of 10% trichloroacetic acid and 1 ml of 0.67% thiobarbituric
acid, followed by heating in a boiling water bath for 30 min.
Thiobarbituric acid reactive substances were determined by
the absorbance at 535 nm and expressed as malondialdehyde
(MDA) equivalents formed. |
Nitric Oxide |
The assay of nitrite in jejunal homogenate was done
according to the method of Berkels et al. [17]. In acid
medium and in the presence of nitrite the formed nitrous
acid diazotises sulphanilamide, which is coupled with
N-(1-naphthyl) ethylenediamine. The resulting azo dye
has a bright reddish-purple color which was measured at
540 nm. |
Quantitative Real-Time PCR |
Trizol (Invitrogen) was used to isolate the total
RNA from mice jejuna. DNA-freeTM kit (Applied
Biosystem, Darmstadt, Germany) was used to digest the
Contaminating genomic DNA. To synthesize cDNA, we
used the QuantiTect® Reverse Transcription kit (Qiagen,
Hilden, Germany). Real time PCR was performed in a Taq-
Man7500 (Applied Biosystems) using the QuantiTectTM
SYBR® Green PCR kit (Qiagen) and the gene-specific
QuantiTectTM primer assay (Qiagen) according to the
manufacturer’s instructions. The primers for mucin genes
(MUC2 and MUC4) and 18S rRNA were purchased from
Qiagen (Hilden, Germany). Following an initial incubation
at 50 °C for 2min, Taq polymerase was activated at 95 °C
for 10 min, 45 cycles followed at 95 °C for 15 s, at 60 °C
for 35 s, and for 30 s at 72 °C. PCR product was measured
as SYBR green fluorescence at the end of the extension
phase. All PCR reactions yielded only a single product of
the expected size as revealed by melting point analysis
and gel electrophoresis. Relative quantitative evaluation
of amplification data was done using Taqman 7500 system
software v.1.2.3f2 (AppliedBiosystems) and the 2−ΔΔc T
method [18]. Expression of the genes was compared to
18S rRNA [19]. |
Statistical Analysis |
Student’s t-test was used to determine significant
differences. Data were represented as means ± SD of
triplicate experiments and P≤0.05 was used to denote
statistical significance. |
Results |
The infection of female C57Bl/6 mice with 106 P. chabaudi
parasitized erythrocytes induced a maximum parasitemia
(about 47%) on day 8 p.i. Light microscopical inspection
of hematoxylin-and-eosin-stained sections revealed that
the epithelial cells of the jejunum of mice infected with
P. chabaudi were injured (Figure 1). The injury was semi
quantified by applying the scoring according to Dommels
et al. [13]. The infection induced a marked inflammatory
injury in the jejunum (Figure 2). In addition, examination
of Alcian blue stained sections (Figure 3) showed a
significant increase (P≤0.05) in goblet cell numbers seen
at the jejuna villi (Figure 4). |
On day 8 p.i. with P. chabaudi parasitized erythrocytes,
there was a significant increase (P≤0.05) in the level of
NO (539 ± 7, μmol/g) and MDA (28±1, nmol/g) (Table
1) while the level of GSH (7±0.1, mg/g) was significantly
(P≤0.05) decreased by the infection (Table 1). |
Quantitative real-time PCR was used to detect changes in
the mRNA levels of mucin genes in the jejunum. Upon
infection with P. chabaudi, there was a significant increase
in the mRNA expression of MUC2 and MUC4 (Figure
5). Gene expression corresponding to MUC2, the main
gel-forming secretory mucin in intestine, significantly
(P<0.01) increased in the jejunum of mice infected with
P. chabaudi when compared to the non-infected animals
(Figure 5). Also, MUC4, another membrane-associated
mucin, was significantly (P≤0.05) up regulated by malaria
infection (Figure 5). This upregulation was approximately
two fold increase when compared to the non-infected
control. |
Discussion |
Our previous studies illustrated that spleen and liver act
as effectors against malaria infection [20, 21]. Up to date,
there is no study investigated the role of the intestine
against malaria infection except Chau et al. [22] who
investigated that increasing L-arginine bioavailability
via oral supplementation can ameliorate malaria-induced
intestinal pathology. |
Getting rid of plasmodial stages is mediated by both
acquired [23] and innate [24] immune responses. The
infected mice were able to heal the induced infection by
P. chabaudi and also, develop immune response against
reinfection. Our results is in agreements with other studies
that found an increase in parasitemia during the phase of
crises [25,26]. |
Oxidative stress markers during infection are found in a
significant increased level compared to the non-infected
controls animals [27-29]. It is know that, oxidative stress
could result from free radical production, this fact also may
be due to increase malondialdehyde which is considere to
be an important lipid peroxidation marker, this indicated
that, oxidative stress is an important process during
parasitic infection [30]. The high Also, the increased level of TBARS, the lipid peroxidation product was seen
in infected red blood cells by P. falciparum, P. vinckei,
P. berghei, and P. chabaudi [31]. In addition, NO has an important role in malaria where increased serum levels
of NO favor parasitemia resolution without affecting the
host. Moreover NO was previously seen as a toxic agent
which is also, responsible for the inflammatory processes
and could indirectly activate cytokines to activate the
immune system [12]. |
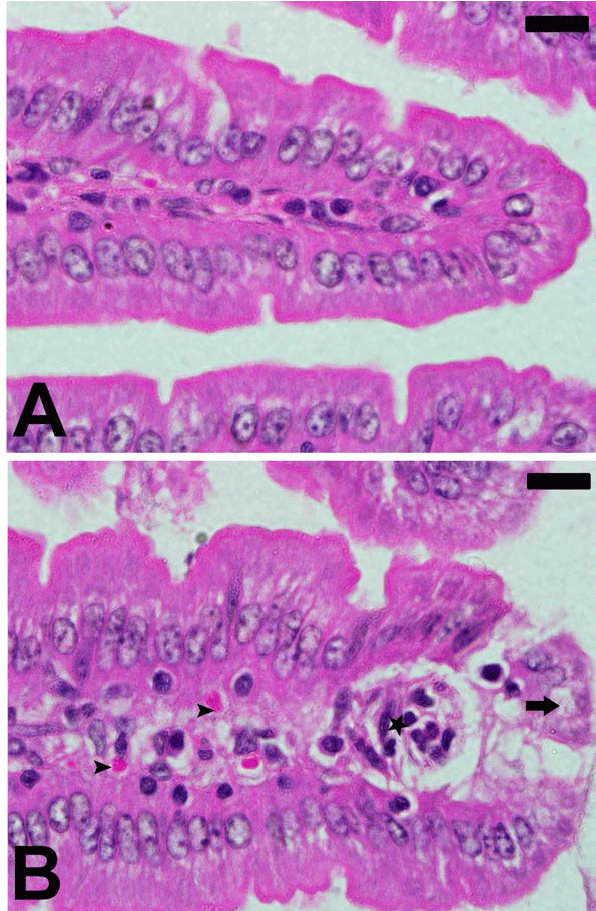 |
Figure 1. Plasmodium chabaudi-induced changes in intestine
histology of C57BL/6 mice. Section from non-infected group
(A) and P. chabaudi infected group (B). Infection induced
inflammation (astar) and cellular vacuolation (arrow). Also,
some parasitized erythrocytes (arrow head) are present.
Sections are stained with hematoxylin and eosin. Scales 25 μm. |
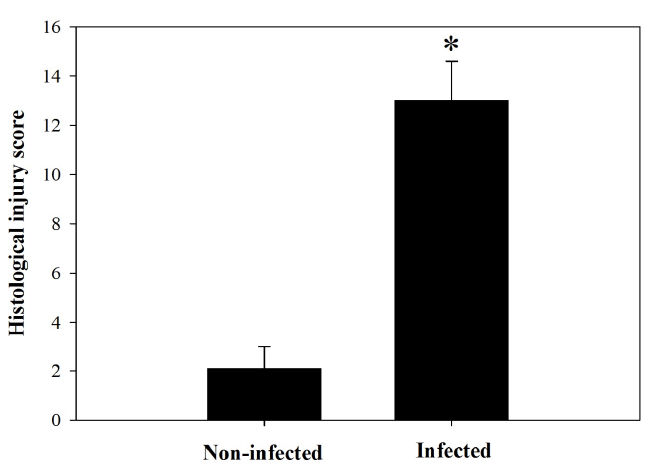 |
Figure 2. Total histological injury scores in intestine of noninfected
and infected mice with P. chabaudi on day 8 p.i.
Scores were calculated according to Dommels et al. (2007).
Values are means ± SD. *Significance against non-infected
group at p ≤ 0.05. |
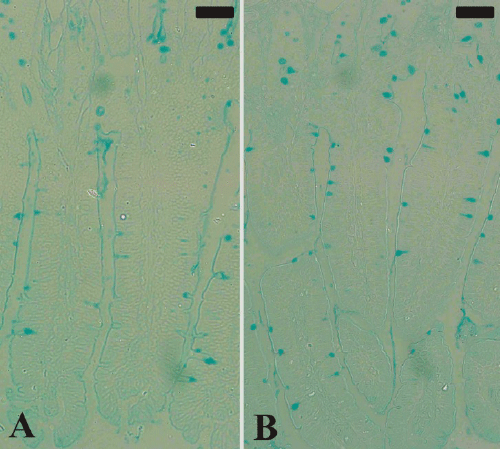 |
Figure 3. Goblet cells in mouse jejunum. Section from noninfected
group (A) and P. chabaudi infected group (B).
Sections were stained with Alcian blue. Bar =50 μm. |
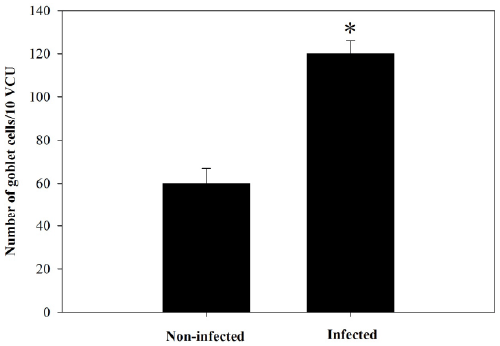 |
Figure 4. Goblet cell number in jejunum of non-infected
and infected mice with P. chabaudi on day 8 p.i. Data were
obtained from Alcian blue-stained sections as shown in
Figure 3. Values are means ± SD. *Significance against oninfected
group at p ≤ 0.05. |
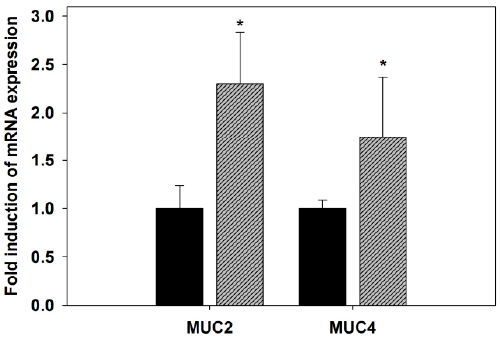 |
Figure 5. Quantitative RT-PCR analysis of MUC2 and MUC4
mRNA in the jejunum of mice. Expression was analyzed in
non-infected and P. chabaudi infected mice on day 8 p.i.,
normalized to 18S rRNA signals, and relative expression is
given as fold increase compared to the non-infected control
mice. Values represent means ± SD. *Significance against
non-infected male mice at p ≤ 0.05. |
Hyperplasia of goblet cells due to malaria has not been
described before. In our model, P. chabaudi infection
is associated with increased goblet cells. However,
hyperplasia of goblet cells has been investigated in a
number of bacterial, viral and parasitic infections [32]. |
Moreover, our results of qRT-PCR, revealed that the
expression of MUC-2 and MUC-4 were significantly
increased due to P. chabaudi infection. It is documented
that, MUC-2 is the first line of innate host defense that
could act to prevent the induced injury due to infection
[33]. In addition, Kim and Ho [33] reported that mucin
plays a critical role in many stages of metastatic processes
of colorectal cancer where colon cancer cell lines that
studied for high capacity for metastasis showed to
upregulate MUC2. Moreover, MUC4 plays important
roles in the carcinogenesis and progression of multiple
human cancers, including pancreatic cancer [34,35]. |
Collectively, studying the intestinal response to P.
chabaudi infection could help in understanding the process
of intestinal oxidative damage as well as the role of mucin
related genes during infection. Further studies are required
to know the mechanism and the pathway by which the
parasite induce these intestinal alterations. |
Acknowledgment |
The authors would like to extend their sincere appreciation to
the Deanship of Scientific Research at King Saud University
for its funding this research group No. (RG-198). |
References |
- Mehlhorn H. Encyclopedic reference of parasitology. 4thEdn. Berlin Springer2014.
- WHO. World Malaria Report, Geneva:World Health Organization2014.
- Seydel KB, Milner DA Jr, Kamiza SB, Molyneux ME, Taylor TE. The distribution and intensity of parasite sequestration in comatose Malawian children. JInfectDis 2006; 194: 208-215.
- Molyneux ME, Looareesuwan S, Menzies IS, Grainger SL, Phillips RE, Wattanagoon Y, Thompson RP, Warrell DA. Reduced hepatic blood flow and intestinal malabsorption in severe falciparum malaria. Am J Trop Med Hyg 1989; 40: 470-476.
- Wilairatana P, Meddings JB, Ho M, Vannaphan S,Looareesuwan S. Increased gastrointestinal permeability in patients with Plasmodium falciparum malaria. Clin Infect Dis 1997; 24: 430-435.
- Specian RD, Oliver MG. Functional biology of intestinal goblet cells. Am J Physiol 1991;260:183-193.
- Corfield AP, Myerscough N, Longman R, Sylvester P, Arul S, Pignatelli M. Mucins and mucosal protection in the gastrointestinal tract: New prospects for mucins in the pathology of gastrointestinal disease. Gut2000; 47: 589-594.
- Ishikawa N, Wakelin D, Mahida YR. Role of T helper 2 cells in intestinal goblet cell hyperplasia in mice infected with Trichinellaspiralis. Gastroenterology 1997; 113: 542-549.
- Hasnain SZ, Evans CM, Roy M, Gallagher AL, Kindrachuk KN, Barron L, Dickey BF, Wilson MS, Wynn TA,Grencis RK, Thornton DJ. Muc5ac: a critical component mediating the rejection of enteric nematodes. J Exp Med 2011; 208: 893-900.
- Metwaly MS, Dkhil MA, Al-Quraishy S. The potential role of Phoenix dactylifera on Eimeriapapillata-induced infection in mice. Parasitol Res 2012;111: 681-694.
- Dkhil MA. Anti-coccidial, anthelmintic and antioxidant activities of pomegranate (Punicagranatum) peel extract. Parasitol Res 2013; 112: 2639-2646.
- Percário S, Moreira DR, Gomes BA, Ferreira ME, Gonçalves AC, Laurindo PS, Vilhena TC, Dolabela MF, Green MD. Oxidative stress in malaria. Int J MolSci 2012; 13: 16346-16372.
- Dommels YE, Butts CA, Zhu S, Davy M, Martell S, Hedderley D, Barnett MP, McNabb WC, Roy NC. Characterization of intestinal inflammation and identification of related gene expression changes in mdr1a(−/−) mice. Genes Nutr 2007; 2: 209-223.
- Allen A, Hutton DA, Leonard AJ, Pearson JP,Sellers LA. The role of mucus in the protection of the gastroduodenal mucosa. Scand J Gastroenterol 1986; 125: 71-78.
- Ellman GL, Tissue sulfhydryl groups. Arch BiochemBiophys 1959;82: 70-77.
- Ohkawa H, Ohishi N, Yagi K. Assay for lipid peroxides in animal tissues by thiobarbituric acid reaction, AnalBiochem 1979; 95: 351-358.
- Berkels R, Purol-Schnabel S, Roesen R. Measurement of nitric oxide by reconversion of nitrate/nitrite to NO. Methods MolBiol 2004; 279: 1-8.
- Livak KJ, Schmittgen TD. Analysis of relative gene expression data using real-time quantitative PCR and the 2 – ˇZ ˇZ C T Method. Methods 2001; 25: 402-408.
- Krucken J, Deli´c D, Pauen H, Wojtalla A, El-Khadragy M, Dkhil MA, Mossmann H, Wunderlich F. Augmented particle trapping and attenuated inflammation in the liver by protective vaccination against Plasmodium chabaudi malaria. Malar J 2009; 8: 54.
- KrückenJ, Mehnert LI, Dkhil MA, El-Kadragy M, Benten WPM, Mossmann H, Wunderlich F. Massive destruction of malaria parasitized red blood cells despite spleen closure. InfectImmun 2005; 73: 6390-6398.
- Wunderlich F, Al-Quraishy S, Dkhil M. Liver-inherent immune system: its role in blood-stage malaria. Front microbial 2014; 5: 559.
- Chau JY, Tiffany CM, Nimishakavi S, Lawrence JA, Pakpour N, Mooney JP, Lokken KL, Caughey GH, Tsolis RM, Luckhart S. Malaria-associated L-arginine deficiency induces mast cell-associated disruption to intestinal barrier defenses against nontyphoidalSalmonella bacteremia. Infect Immun 2013; 81: 3515-3526.
- Celada A, Cruchaud A, Perrin LH. Phagocytosis of Plasmodium falciparum-parasitized erythrocytes by human polymorphonuclear leukocytes. J Parasitol 1983; 9: 49-53.
- Serghides L, Kain KC. Peroxisome proliferator-activated receptor gamma-retinoid X receptor agonists increase CD36-dependent phagocytosis of Plasmodium falciparumparasitized erythrocytes and decrease malaria-induced TNF alpha secretion by monocytes/macrophages, J Immunol 2001; 166: 6742-6748.
- Helmby H, Jönsson G, Troye-Blomberg M. Cellular changes and apoptosis in the spleens and peripheral blood of mice infected with blood-stage Plasmodium chabaudichabaudiAS. Infect Immun 2000; 68: 1485-1490.
- Dkhil MA. Apoptotic changes induced in mice splenic tissue due to malaria infection. J microbial Immunol Infect 2009; 42: 13-18.
- Clark IA, Hunt NH. Evidence for reactive oxygen intermediates causing hemolysis and parasite death in malaria. Infect Immun 1983; 39: 1-6.
- Guha, M, Kumar S, Choubey V, Maity P, Bandyopadhyay U. Apoptosis in liver during malaria: Role of oxidative stress and implication of mitochondrial pathway. FASEB J 2006;20: 439-446.
- Sohail M, Kaul A, Raziuddin M, Adak T. Decreased glutathione-S-transferase activity: Diagnostic and protective role in vivaxmalaria. ClinBiochem 2007;40: 377-382.
- Cabrales P, Zanini GM, Meays D, Frangos JA, Carvalho LJM. Nitric Oxide protection against murine cerebral malaria is associated with improved cerebral microcirculatory physiology. J Infect Dis 2011; 203: 1454-1463.
- Das BS, Nanda NK. Evidence for erythrocyte lipid peroxidation in acute falciparum malaria.Trans R Soc Trop Med Hyg 1999; 93: 58-62.
- KimJJ, Khan WI. Goblet cells and mucins: Role in innate defense in enteric infections. Pathogens 2013; 2:55-70.
- Kim YS, Ho SB. Intestinal Goblet Cells and Mucins in Health and Disease: Recent Insights and Progress. CurrGastroenterol Rep 2010; 12: 319-330.
- Hollingsworth MA, Swanson BJ. Mucins in cancer: protection and control of the cell surface. Nat Rev Cancer 2004; 4: 45-60.
- Chaturvedi P, Singh AP,Batra SK. Structure, evolution, and biology of the MUC4 mucin, FASEB J 2008; 22: 966-981.
|