Keywords
|
Occlusal hypofunction, Vascular Endothelial Growth Factor (VEGF), Periodontal Ligament (PDL). |
Introduction
|
Occlusal stimuli are essential factor for maintaining the structural integrity of the Periodontal ligament (PDL), which has a functional structure for occlusal loads [1]. Teeth without occlusal stimuli are referred to as hypofunctional teeth. Numerous atrophic changes have been reported in the PDL of hypofunctional teeth following the lack of occlusal function, including thickening of periodontal collagen fibers, disorientation of the collagen fibers, narrowing of periodontal space, and loss of alveolar bone, [2-6]. Using three-dimensional microcomputed tomography analyse, we showed that the PDL structure in mice may be affected by disuse atrophy due to defects in occlusal function, and this atrophy may be recovered by regaining occlusal stimuli [7,8]. In orthodontic practice, previous studies demonstrated that the severity of root resorption, examined radiographically, was greater in open-bite cases, which had many hypofunctional teeth, than in deep-bite case before and after orthodontic treatments [9]. We also showed that the prevalence of root resorption and teeth with an abnormal root shape in open-bite patients was significantly greater in hypofunctional teeth than in normal functional teeth [10]. A close relationship exists between periodontal blood vessels and occlusal stimuli. This discovery includes a reduction in the diameter and number of periodontal blood vessels [11]. Several studies using occlusal recovery models observed the revealed widening of blood vessels in the PDL following the application of occlusal stimuli [12-14]. Vascular endothelial growth factor (VEGF) is the primary mediator of angiogenesis [15] and serves various biological functions, such as increasing vascular permeability [16]. It is also involved in bone resorption [17-19]. Therefore, VEGF may play an important role in periodontal remodeling after occlusal stimuli. Recent studies reported that occlusal hypofunction induced a decrease in the expression of VEGF and FGF (Fibroblast Growth Factor), whereas the expression of FGF was observed in the recovery process [20,21]. However, the structure of the PDL and expression of VEGF have not yet been examined in the occlusal recovery process. Therefore, the aim of study was to determine whether occlusal hypofunction and its recovery influenced the structure of the PDL and expression of VEGF in mice. |
Materials and Methods
|
Animals and the experimental model |
5-week-old C57BL/6J mice (Jackson Laboratory, Bar Harbor, Me, USA) were used and randomly divided into three groups: two experimental groups and one Control group (CG; n=4). The two experimental groups included a Hypofunctional group (HG; n=4) and Recovery group (RG). The appliance used in the experimental groups consisted of a metal cap made of band material (Tomy International Co., Fukushima, Japan) and an anterior-bite plate made of a new ST lock base (Dentsply- Sankin, Tokyo, Japan) bonded with composite resin (ClearfilMajesty LV; Kuraray Co. Ltd., Okayama, Japan) to the maxillary and mandibular incisors, respectively [7,13] (Figure 1A and 1B). In CG and HG, mice were killed at 16 weeks of age. In RG, 16 mice, from which the appliance had been removed 7 weeks after its attachment, were killed at 0, 7, 14, and 28 days after removal (n=4, respectively). HG were then subjected to soft X-ray radiography to confirm occlusal conditions at 12 weeks of age. The experimental program and procedures are summarized in Figure 1C. Animals were fed a powder diet (Rodent Diet CE-2; Japan CLEA Inc., Tokyo, Japan) and had free access to drinking water under a 12-h light/ dark environment at a constant temperature of 23°C. |
Tissue preparation |
Mice were anesthetized by an injection of chloral hydrate and perfused with a 4% periodate–lysine–paraformaldehyde fixative solution (pH 7.4) through the descending aorta. Maxillae were decalcified in 14% EDTA (pH 7.4) for 28 days and then embedded in paraffin. Sections (5-μm thick) were cut horizontal to the maxillary incisors. These sections performed HE staining and immunohistochemistry staining of VEGF in turn. Then each section’s photographs were taken by microscope. |
Immunohistochemical staining |
After deparaffinization, sections that included the root canal were treated with 3% hydrogen peroxide in absolute methanol to block endogenous peroxidase. Sections were then rinsed and immunostained with a 1:150 dilution of primary anti-rat VEGF chicken polyclonal antibodies (Abcam Inc., Boston, MA), followed by an anti-chicken secondary IgG antibody (Abcam), and then an anti-rabbit secondary IgG antibody (Hystfine simple stain rat MAX-PO(R); Nichirei, Tokyo, Japan). Immunoreactive sites were visualized by 3,3'- diaminobenzidine (DAB). Counterstaining was performed using hematoxylin. Sections from each group incubated without the primary antibody were used as negative controls. |
Analysis of the PDL area |
The PDL area in the distobuccal root was analyzed after typical serial sections, including the longest root canal, which were selected and measured by a blinded examiner (n=4, respectively) (Figure 2A). Each of the sections was measured with an image scanner connected to a personal computer with image-analyzing program (BZ analyzer, Keyence, Tokyo, Japan). |
Quantitative analysis |
In immunohistochemically stained sections, the middle onethird palatal aspect of the distobuccal root was photographed using an optical microscope (Biozero, Keyence). Quantitative images were measured by a blinded examiner using image analysis software (BZ analyzer, Keyence). The number of VEGF-positive PDL cells was counted within the rectangular area (100 × 300 μm) by a blinded examiner (n=4, respectively) (Figure 2B). |
Statistical analysis |
The mean body weight (gt) and number immunopositive PDL cells per area (mm) in the mouse PDL were expressed as means ± standard error of the mean. To determine the significance of differences among the 3 groups, a performed repeated one-way analysis of variance and Tukey–Kramer test were per formed using Statview. (Abacus Concepts Inc., Berkeley, CA). The level of significance was set at level P<0.05. |
Results
|
No significant differences were observed in mean body weights between the 3 groups (Table 1). However, the PDL area was high and significantly larger in CG and RG than in HG (P<0.01), where it was similar to that in RG and CG (Figure 3A). In the recovery process, the PDL increased from days 0, peaked at 7 days and the decreased from days 7 to 28 (Figure 3B). Significant differences in the PDL area were observed between days 0 and 7 (P<0.01), days 7 and 14 (P<0.05), and days 7 and 28 (P<0.01). Histologically, bone resorption lacunae along the alveolar bone were the largest at 7 days (Figure 4A-4F). |
Expression of VEGF |
The number of VEGF-positive cells was significantly larger in CG and RG than in HG (P<0.01), while the number of these cells was similar in RG and CG (Figure 5A). In the recovery process, the number of VEGF-positive cells was significantly higher after 1 day and peaked on day 7 (P<0.01; Figure 5B). After 7 days, the number of cells significantly decreased until 28 days (P<0.01). Immunohistochemically, the enhanced expression of VEGF was observed in alveolar bone-lining cells, fibroblastic cells, and endothelial cells. The expression of VEGF peaked at 7 days and then decreased from days 7 to 28 (Fig. 6A-6F). |
Discussion
|
The present study was conducted to investigate the influence of occlusal stimuli on the PDL area and expression of VEGF in the PDL. We established experimental hypofunctional conditions in the molar region by using a bite-raising technique [14]. This method made it possible to reestablish occlusion following the removal of appliances. This model of opposite molar extraction was previously used to produce occlusal hypofunction, however, difficulties were associated with recovering normal occlusion. In our experimental model, occlusal hypofunction was induced in the molar area, which caused hypofunctional changes in the PDL. Our results showed that changes in occlusal conditions affected the expression of VEGF, This which may be explained by the complex relationship between occlusal stimuli and the expression of VEGF. VEGF is known to play an important role in angiogenesis [16,19,20]. Previous studies demonstrated that occlusal hypofunction induced a decrease in the expression of VEGF [21-23], which is inconsistent with the results of the presents study. Moreover, several studies using occlusal recovery models revealed the widening of blood vessels in the PDL following the application of occlusal stimuli [12-14]. These findings suggest that VEGF has an important regulatory role in angiogenesis and blood vessel expansion as a mediator of occlusal stimuli. Occlusal stimuli are essential factor for maintaining the structural integrity of the PDL, the function of which is to apply suitable occlusal pressure [1]. Numerous atrophic changes have been reported in the PDL of hypofunctional teeth [2-6], and this atrophy may be recovered after the reapplication of occlusal stimuli in mice [7]. In this study, we histologically showed that the PDL area in HG increased following appliance of and removal and was similar to that in CG. In the recovery process, the PDL area increased from days 0 to 7 and decreased from days 7 to 28. Furthermore, the PDL area peaked after 7 days and the size of alveolar bone resorption lacunae was the largest at 7 days. The number of VEGF-positive cells in the PDL also peaked at 7 days, after which the number of cells decreased to the levels observed in CG. The enhanced expression of VEGF was also observed in alveolar bone-lining cells, fibroblastic cells, and endothelial cells. VEGF is also involved in bone resorption [17,18], playing an important role in the differentiation of mesenchymal cells into osteoblasts [21,22]. These findings indicate that the expression of VEGF induced osteoclasts, and that alveolar bone was resorbed, there by inducing increases in the PDL area until day 7. After 7 days, the expression of VEGF decreased markedly. Therefore, VEGF may play an important role both angiogenesis and in bone remodeling. |
Conclusions
|
The results of the present study suggest that occlusal stimuli regulate the expression of VEGF in PDL cells, and that this growth factor lead to alveolar bone remodeling in PDL. |
Tables at a glance
|
 |
Table 1 |
|
|
Figures at a glance
|
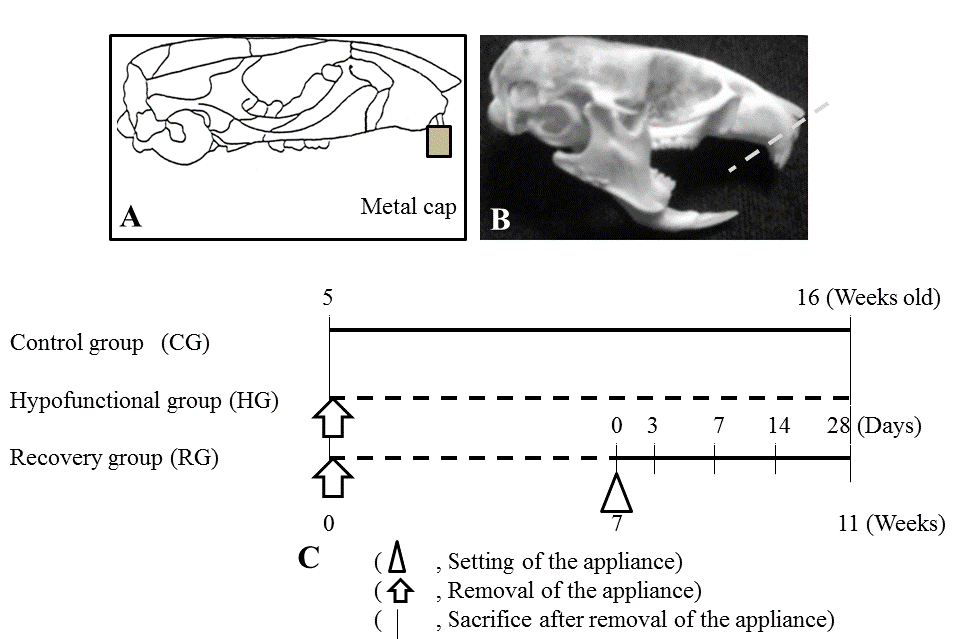 |
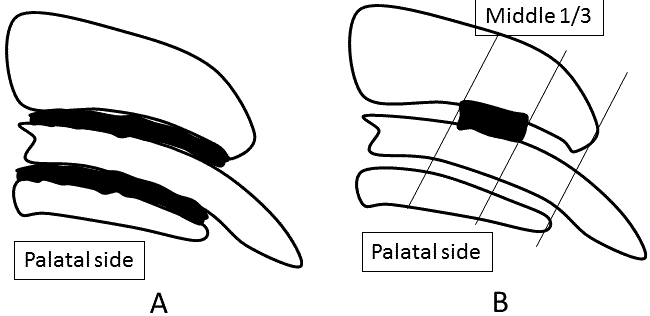 |
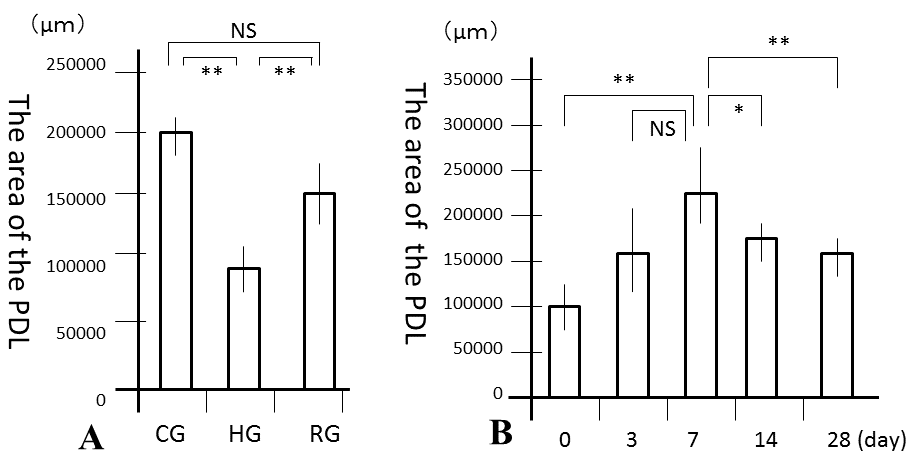 |
Figure 1 |
Figure 2 |
Figure 3 |
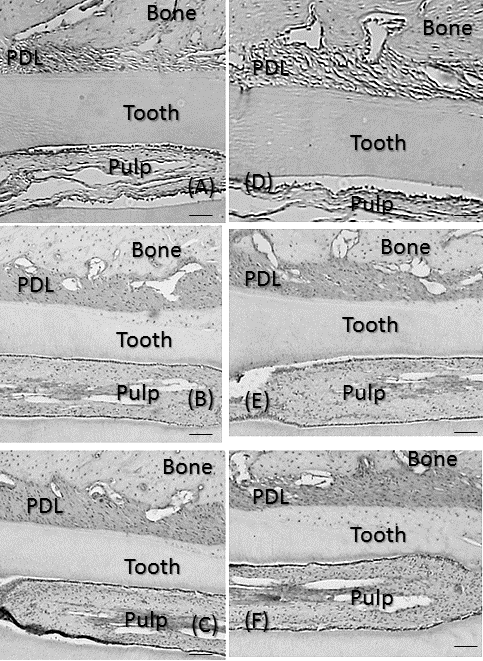 |
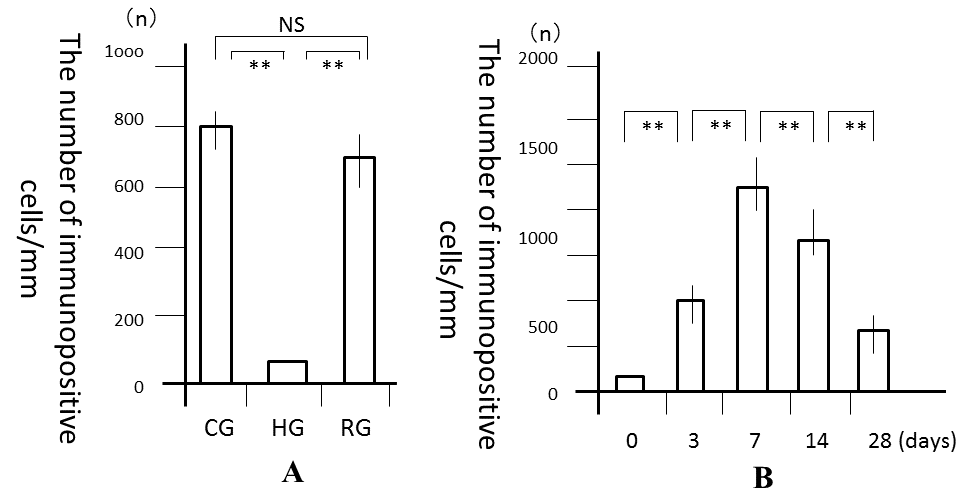 |
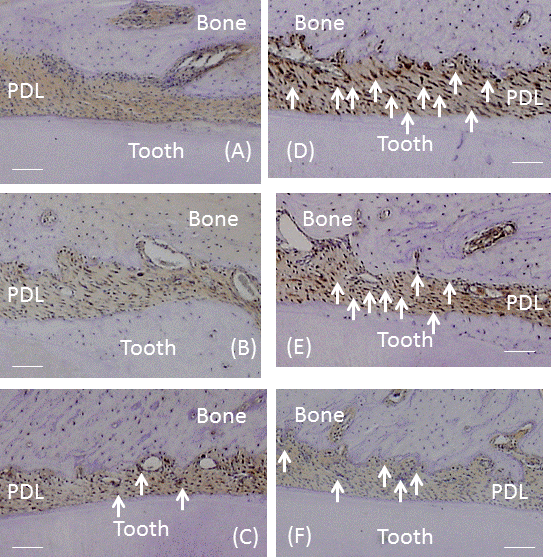 |
Figure 4 |
Figure 5 |
Figure 6 |
|
|
References
|
- Muramoto T, Takano Y, Soma K. Time-related changes in periodontal mechanoreceptors in rat molars after the loss of occlusal stimuli. Arch Histol Cytol 2000; 63: 369-380.
- Cohn SA. Disuse atrophy of the periodontium in mice. Arch Oral Biol. 1965; 10: 909-919.
- Cohn SA. Disuse atrophy of the periodontium in mice following partial loss of function. Arch Oral Biol 1966; 11: 95-105.
- Levy GG, Mailland ML. Histologic study of the effects of hypofunction following antagonist tooth extraction in the rat. J Periodontol 1980; 51: 393-399.
- Shimizu Y, Hosomichi J, Kaneko S, Shibutani N, Ono T. Effect of sympathetic nervous activity on alveolar bone loss induced by occlusal hypofunction in rats. Arch Oral Biol. 2011; 56: 1404-1411.
- Bernick S. The organization of the periodontal membrane fibres of the developing molars of rats. Arch Oral Biol 1960; 2: 57-63.
- Motokawa M, Terao A, Karadeniz EI, Kaku M, Kawata T. Effects of longterm occlusal hypofunction and its recovery on the morphogenesis of molar roots and the periodontium in rats. Angle Orthod. 2013; 83: 597-604.
- Motokawa M, Kaku M, Matsuda Y, Kojima S, Sumi C. Effects of occlusal hypofunction and its recovery on PDL structure and expression of VEGF and bFGF in rats. Clin Oral Invest. 2014; 11: 1310-1316.
- Harris EF, ButlerML. Patterns of incisor root resorption before and after orthodontic correction in cases with anterior open bites. AmJ Orthod Dentofac Orthop 1992; 101:112-119.
- Motokawa M, Terao A, Kaku M, Kawata T, Gonzales C. Open bite as a risk factor for orthodontic root resorption. Eur J Orthod. 2013; 35: 790-795.
- Tanaka A, Iida J, Soma K. Effect of hypofunction on the microvasculature in the periodontal ligament of the rat molar. Orthod Waves 1998; 57: 180-188.
- Koike K. The effects of loss and restoration of occlusal function on the periodontal tissues of rat molar teeth-histopathological and histometrical investigation. Periodontol 1996; 38: 1-19.
- Saeki M. Experimental disuse atrophy and its repairing process in the periodontal membrane. J Stomatol Soc Jpn 1959; 26: 317-347.
- Suhr ES, Warita H, Iida J, Soma K. The effect of occlusal hypofunction and its recovery on the periodontal tissues of the rat molar: ED1 immunohistochemical study. Orthod Waves 2002; 61:165-172.
- Leung DW, Cachianes G, KuangWJ, Goeddel DV, Ferrara N. Vascular endothelial growth factor is a secreted angiogenic mitogen. Science (New York, NY) 1989; 246: 1306-1309.
- Senger DR, Galli SJ, Dvorak AM, Perruzzi CA, Harvey VS, Dvorak HF. Tumor cells secrete a vascular permeability factor that promotes accumulation of ascites fluid. Science (New York, NY) 1983; 219: 983-985.
- Aldridge SE, Lennard TW, Williams JR, Birch MA. Vascular endothelial growth factor acts as an osteolytic factor in breast cancer metastases to bone. Br J Cancer 2005; 92: 1531-1537.
- Niida S, Kaku M, Amano H, Yoshida H, Kataoka H. Vascular endothelial growth factor can substitute for macrophage colony-stimulating factor in the support of osteoclastic bone resorption. J Exp Med 1999; 190: 293-298.
- Kawata T, Kohno S, Kaku M, Fujita T, Ohtani J. Expression of vascular endothelial growth factor on neovascularization during experimental tooth movement by magnets. Biomed Res 2011; 22: 248-253.
- Faitova J. Fibroblast growth factor-2. Cesk Fysiol/Ustred Biol 2004; 53: 92-101.
- Gospodarowicz D. Fibroblast growth factor. Chemical structure and biologic function. Clin Orthop Relat Res 1990; 257; 231-248.
- Boonpratham S, Kanno Z, Soma K. Occlusal stimuli regulate interleukin-1 beta and FGF-2 expression in rat periodontal ligament. J Med Dental Sci 2007; 54: 71-77.
- Usumi-Fujita R, Hosomichi J, Ono N, Shibutani N, Kaneko S. Occlusal hypofunction causes periodontal atrophy and VEGF/VEGFR inhibition in tooth movement. Angle Orthod 2013; 83: 48-56.
|